Chemicals and instrumentation
All of the chemicals and reagents were used without additional purification; they were purchased from Aldrich (Sigma–Aldrich, St. Louis, MO, USA). 1H and 13C NMR spectra were recorded on Gemini Varian-VXR-unity (400, 300 MHz) instrument. Chemical shifts (δ) are reported in ppm downfield from internal TMS standard.
Synthesis of C– 4- Hydroxyphenylcalix[4] resorcinarene (HPCR)
The HPCR (Fig. 1) was resynthesized as reported previously by Al-Trawneh et al.12. The complete procedure and structural elucidation of HPCR compound have already been provided in our prior study13. HPCR was resynthesized by a single-step organic reaction (Fig. 2). Briefly, an ice-cooled solution of resorcinol (2.18 g) and HCl (10 M, 3 mL) was mixed with p-Hydroxybenzaldehyde (2.42 g) dissolved in ethanol (40 ml). The resultant mixture was stirred at room temperature overnight. Afterward, a light pink precipitate was filtered and rinsed several times with 50% aqueous ethanol. The obtained RESORCINOL was left overnight in the dark to fully dry. The collected amount was 2.35 g, m.p = 290 °C, (decomp).
![Cheminformatics-based design and biomedical applications of a new Hydroxyphenylcalix[4] resorcinarene as anti-cancer agent Cheminformatics-based design and biomedical applications of a new Hydroxyphenylcalix[4] resorcinarene as anti-cancer agent](https://media.springernature.com/lw685/springer-static/image/art%3A10.1038%2Fs41598-024-82115-1/MediaObjects/41598_2024_82115_Fig1_HTML.png)
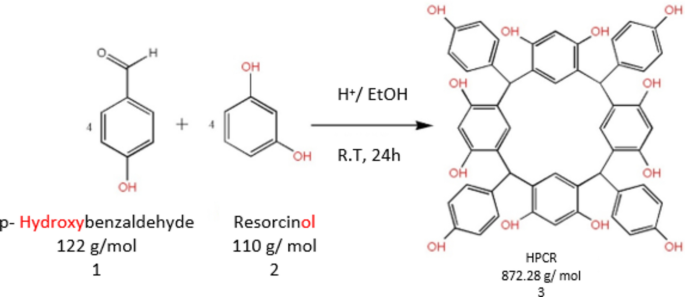
Synthesis of C-4-Hydroxyphenylcalix[4] resorcinarene (HPCR).
1H NMR (400 MHz, DMSO-d6): δ = 5.43 and 5.52 (s, 4H, H-7, 8, 31, 38), 6.08 and 6.10 (s, 4H. H-3, 13, 36, 44), 6.32 and 6.43 (d, J3 = 8.0, 8.0 Hz, 8H, H-19, 21, 26, 28, 47, 49, 60,62), 6.49 and 6.50 (s, 4H, H-6, 10, 33, 41), 6.48 and 6.64 (d, J 3 = 8.0, 8.0 Hz, 8H, H-18, 22, 25, 29, 46, 50, 59, 63), 8.38 and 8.43 (s, 4H, H-23, 30, 51, 64), 8.64 and 8.87 (s, 4H, H-15, 16, 22, 25, 50, 52, 53, 55).
13C NMR (100 MHz, DMSO-d6): δ = 40.5 (C-7, 8, 31, 38), 101.9 (C-3, 13, 36, 44), 113,9 (C-19, 21, 26, 28, 47, 49, 60, 62), 120.9 (C-1, 5, 9, 11, 32, 34, 39, 42), 129.5 (C-6, 10, 33, 41), 129.7 (C-18, 22, 25, 29, 46, 50, 59, 63), 135.9 (C- 17, 24, 40, 58), 152.5 (C-2, 4, 12, 14, 35, 37, 43, 45), 154.4 (C-20, 27, 48, 61).
X-ray crystallographic analysis indicated that C-4-Hydroxyphenylcalix[4]resorcinarene crystallizes were in the triclinic P-1 (2). It is important to note that the alcohol groups contribute to the production of intermolecular hydrogen bonds in the HPCR compound, which maintains the stability of the crystal network. Figure 3 shows the projections of the structure along the a, b and c axis. with the hydrogen bonds.
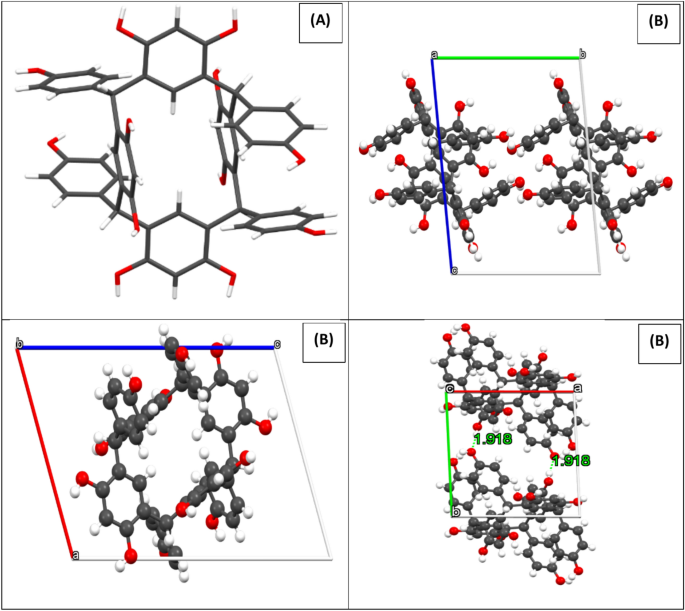
(A) The HPCR structure (capped sticks), and (B) Projection of the structure along a, b and c axis. Black, red, and snowballs correspond to carbon, oxygen and hydrogen atoms.
Cell culture
The breast cancer (MCF-7), non-small cell lung cancer (A549), glioblastoma (U-87 MG) and normal human dermal fibroblast (HDF) cell lines were obtained from American Type Culture Collection (ATCC, Manassas, United States). All culture mediums, heat-inactivated fetal Calf serum (FCS), L-glutamine, sodium pyruvate and penicillin- streptomycin antibiotics (P-S) were obtained from Capricorn Scientific GmbH, Ebsdorfergrund, Germany. The MCF-7 and A549 cell lines were cultured and maintained in a Roswell-Park Memorial Institute medium (RPMI 1640) containing 2 mM of L-glutamine, 10% (v/v) of FCS, 1 mM of sodium pyruvate and 1% of (P-S). Moreover, the (U-87 MG) and (HDF) were cultured and maintained in Dulbecco’s Modified Eagle Medium (DMEM) containing 2 mM of L-glutamine, 10% (v/v) of FCS, 1 mM of sodium pyruvate and 1% of (P-S). All these cell lines were kept in the tissue culture incubator at 37 ºC in a 5% CO2 humidified atmosphere.
Cytotoxicity assay
Thiazolyl Blue Tetrazolium Bromide (MTT) assay was performed to determine the anti-proliferative activity of the HPCR, as mentioned previously14,15,16. After harvesting of cells, cells were inoculated in a round bottom 96-well plate (Corning, USA) at a density of 1 × 104 cells/ml and incubated overnight at 37 °C in a 5% CO2 atmosphere, to allow cell adherence. Cells were then incubated with HPCR compound (1, 50, 100, 200, 400, 600, 800, and 1000 μM/ mL). The anti-cancer drug cisplatin (Sigma-Aldrich Chemie GmbH, Germany) was used as a positive control with different concentrations of 1, 10, 50, and 100 μM/mL in all experiments. After 96 h of cell treatment, the medium was aspirated, and 0.2 mL MTT at a concentration of 5 mg/mL (Sigma-Aldrich Chemie GmbH, Germany) was added to each well for 4 h. Finally, the supernatant was removed and the formed formazan crystals were dissolved in 0.15 mL Dimethyl sulfoxide (DMSO, Sigma-Aldrich Chemie GmbH, Germany). After that, the absorbance of formazan solutions was measured at 550 nm using BioTek Synergy HTX multimode readers (Agilent Technologies, CA, USA).
The Half-maximum inhibitory concentration (IC50) to HPCR was used to determine the 50% cell growth inhibitory effect of HPCR against various normal and cancer cell lines. The IC50 was calculated by non-linear regression method for each cell line via GraphPad Prism 7 Software, California, USA. Additionally, the degree of HPCR to induce selective cytotoxicity toward cancer cells was determined by the selectivity index (SI). It is the ratio of the inhibitory effect of HPCR on human normal cells (HDF) to the inhibitory effect on cancer cells (A549, U-87 MG, and MCF-7). SI more than 3, indicates that HPCR has selectivity towards particular cancer cells than normal cells17,18.
Morphological analysis
Morphological analysis was performed with some modifications as previously stated19,20,21. Cells were inoculated at the density of 1 × 104 cells/ml (180 μL/well) in a flat bottom 96-well plate (Corning, USA). After overnight incubation to allow cell adherence, cells were treated with HPCR and cisplatin at the same concentrations as described earlier. Cells were also treated with HPCR and cisplatin at the respective IC50 for each cell line. Afterward, the treated cells were stained with hematoxylin after fixation with ice-cold methanol (4 °C) for 30 min. Plates were viewed under a Zeiss Axiovert 40C inverted microscope and digital images were captured using ToupCam digital camera.
In silico predication of toxicity
The online ProTox-II webserver was utilized to assess the toxicity of the synthesized HPCR (https://tox.charite.de/protox3/index.php?site=compound_input, accessed on 20 August 2024). This tool predicts acute oral toxicity in rodents as function of median lethal dose (LD50) and classifies compounds into six toxicity classes ranging from class I (very toxic) to class VI (non-toxic). It further incorporates models for prediction of organ toxicity, different toxicity endpoints, toxicological targets and pathways, compound metabolizing enzymes and finally molecular initialling events behind toxic effect22,23.
Statistical analysis
The statistical packages for social sciences (SPSS) program version 19 was used to analyze all variables. For each experimental run, the data were presented as average ± standard deviation (SD). The statistical significance of the differences in the means of the three experiments was assessed using the t-test. If the p-value was less than 0.05, a statistical difference was regarded as significant.
Technique of molecular operating environmental‑docking (MOE)
The MOE docking approach module (Vs. 2015) represents the latest software in the realm of docking simulation techniques, specifically designed to assess the inhibitory potential against cancerous proteins24. The selected proteins for evaluation included cancer cell lines (U-87, MCF-7, A549) and the human dermal fibroblast cell line (HDF). The respective protein structures were obtained from the Protein Data Bank (PDB: 3RJ3), (PDB: 7AXD), (PDB: 6DUK), and (PDB: 1CGL). The simulation technique was applied to C- 4- Hydroxyphenylcalix [4] resorcinarene (HPCR) and the standard drug Cisplatin obtained from the PubChem database (http://pubchem.ncbi.nlm.nih.gov), commencing with orientation and progressing to optimization for the tested compound25. Pre-optimization involved configuring the compounds by adding hydrogen atoms, atomic charges, and potential energies, with adjustments made using the MMFF94x force field during energy minimization26. The orientation of proteins was a key focus, beginning with the placement of hydrogen atoms over receptors after removing water molecules. The subsequent stages included connecting receptor types, fixing potential energies, and conducting site-finder analysis over the line helix of protein amino acids. Dummies were adjusted over alpha-sites in the protein, paving the way for the initiation of the docking process. The docking simulation process varied in duration for all tested compounds, but each process comprised an average of 30 poses. Various features, such as ligand type, receptors, interaction type, H-bond length, and energy content, were recorded for the docked complexes27,28. Furthermore, interaction patterns and surface maps were extracted to validate comparative features.
Density functional theory approach
Several theoretical approaches have been used to study the local and global reactivity of various organic and inorganic compounds in different fields such as synthesis, medicine corrosion…..etc.29. To examine these features, density functional theory (DFT), has been essential. Furthermore, to optimize the structure of the anticancer drug HPCR, we applied the basic set of digital double polarization plus (DNP) and GGA/PBE functions in Materials Studio software using DMol3 methodologies. HOMO and LUMO energies, energy gap (ΔEgap), electron affinity (EA), ionization potential (IP), electronegativity (χ), percentage of electrons transported (ΔN), and dipole moment characteristics (μ), are among the theoretical parameters that were calculated using Eqs. 1 to 7 refs.30,31. In addition, these theoretical tools facilitated the identification of several isosurfaces using Multiwfn, VMD, and Gnuplot, including the optimal structure, HOMO and LUMO isosurfaces, as well as ELF, LOL, NCI, and RDG isosurfaces. We used Hirshfeld population analysis to calculate Fukui indices (Fukui ( +) and Fukui (−)). To predict electrophilic and nucleophilic sites. These parameters were calculated using Eqs. 8 and 9 ref.32. Additionally, to investigate the interactions between the anticancer drug HPCR and four proteins (PDB: 3RJ3, PDB: 7AXD, PDB: 6DUK, and PDB: 1CGL), we optimized their structures using the Forcite technique in Materials Studio software33.
$$\Delta E_{gap} = E_{LUMO} – E_{HOMO} = \,{\text{IP}} – {\text{EA}}$$
(1)
$${\chi }_{inh}=\frac{IP+EA}{2}$$
(4)
$${\eta }_{inh}=\frac{{\Delta E}_{gap}}{2}$$
(5)
$$\mu =\frac{{E}_{LUMO}+{E}_{HOMO}}{2}$$
(6)
$${\Delta N}_{max}=\frac{-\mu }{{\eta }_{inh}}$$
(7)
$${\text{f}}_{{\text{k}}}^{ + } = {\text{q}}_{{\text{k}}} \left( {{\text{N }} + { 1}} \right) – {\text{q}}_{{\text{k}}} \left( {\text{N}} \right)$$
(8)
$${\text{f}}_{{\text{k}}}^{ – } = {\text{q}}_{{\text{k}}} \left( {\text{N}} \right) – {\text{q}}_{{\text{k}}} \left( {{\text{N}} – {1}} \right)$$
(9)
The electron concentrations on atom k that correspond to N+1, and N−1 are represented by the symbols qk (N+1), qk (N), and qk (N−1). Systems with N+1, N, and N−1 electrons, respectively.