AI headshot generators have come a long way. Few years ago, they produced bizarre, uncanny results that were fun to look at but far from usable. Now? They’re so realistic you have to double-check to realize they’re not real photos.
You don’t need to book a pricey photographer or spend hours in a studio anymore. Just upload some selfies, let the AI work its magic, and boom—you’ve got professional-quality photos ready to boost your social media profile or resume.
These headshots can make a huge difference. And the best part?
You don’t need to deal with awkward posing or hefty photography bills.
If you care about making a great first impression online, these tools are an easy win.
My Top Picks for AI Headshot Generators
#1 PortraitPal
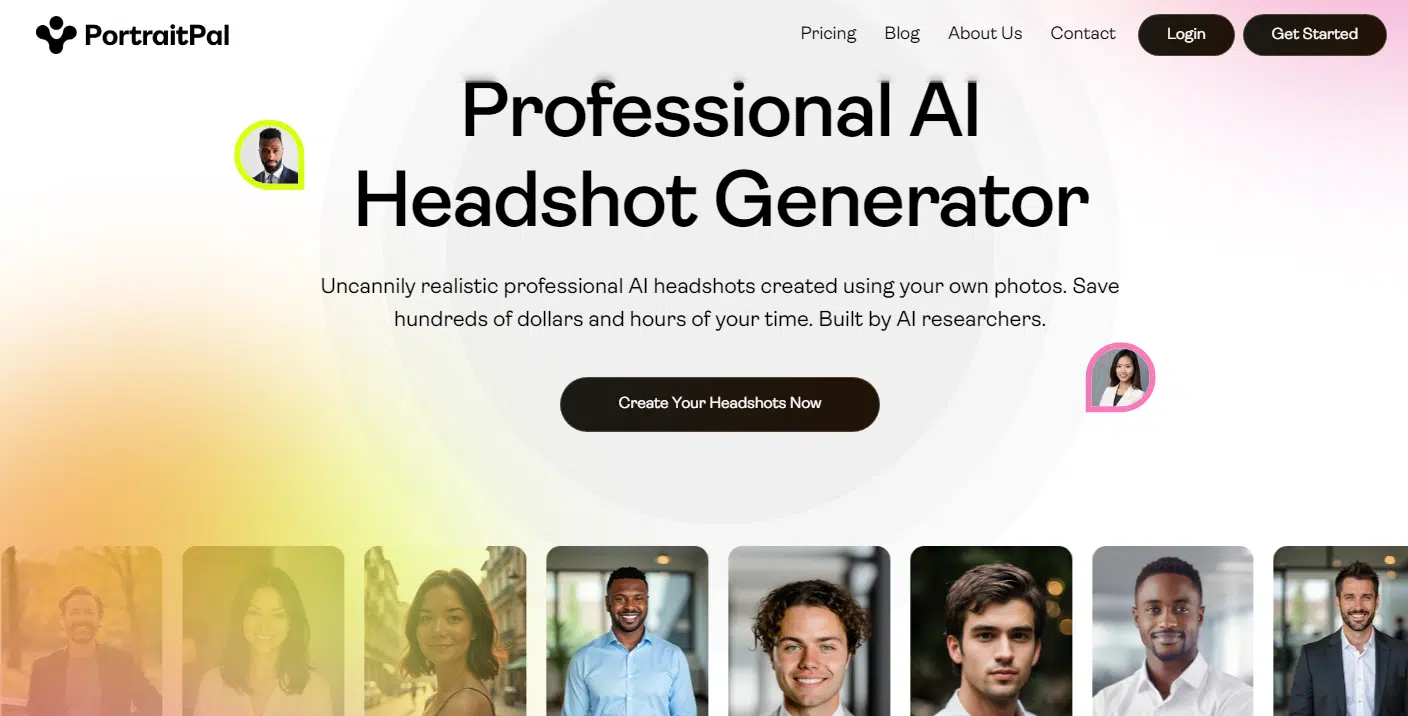
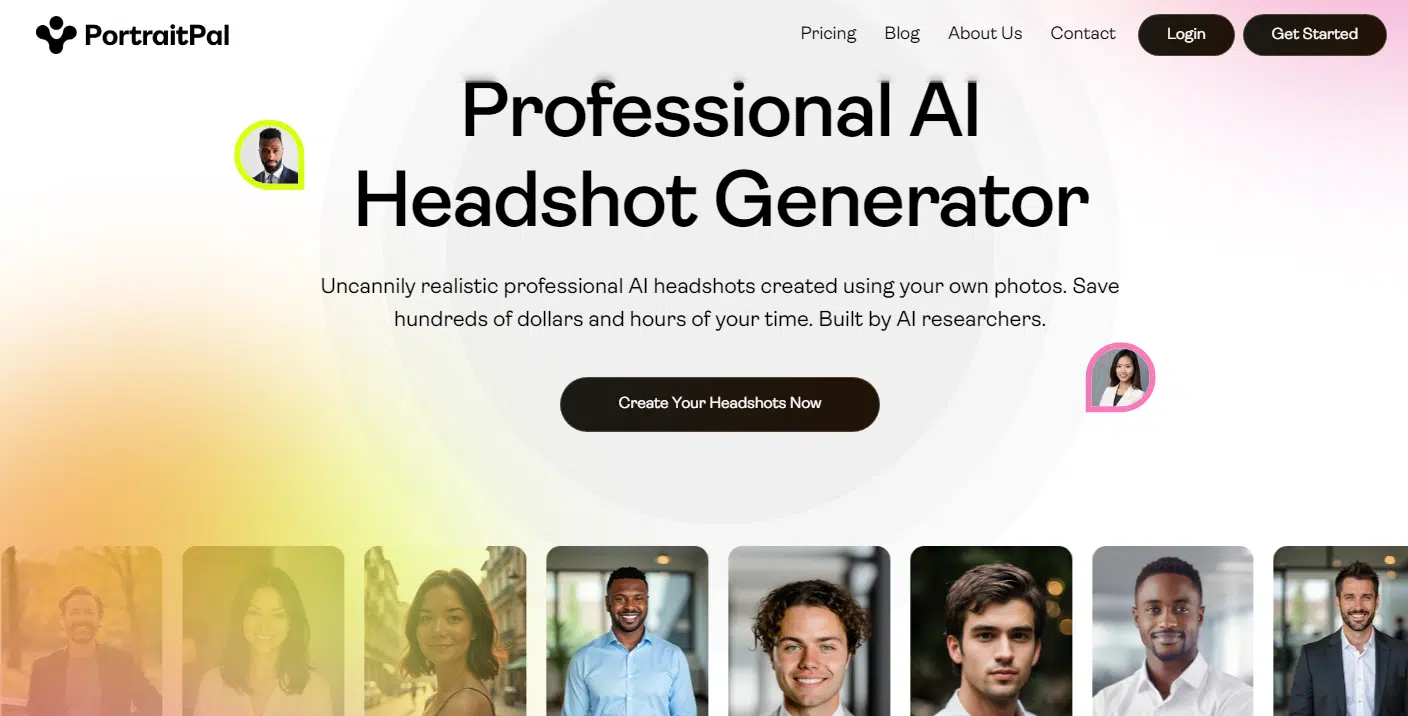
Portrait Pal is a solid go-to if you’re looking for quick, high-quality results without any fuss. The headshots it generates are impressively realistic, with sharp details and backgrounds that look clean and professional—none of that blurry, artificial vibe you might get from other tools. You’d be hard-pressed to figure out these photos were made by AI, even if you looked closely.
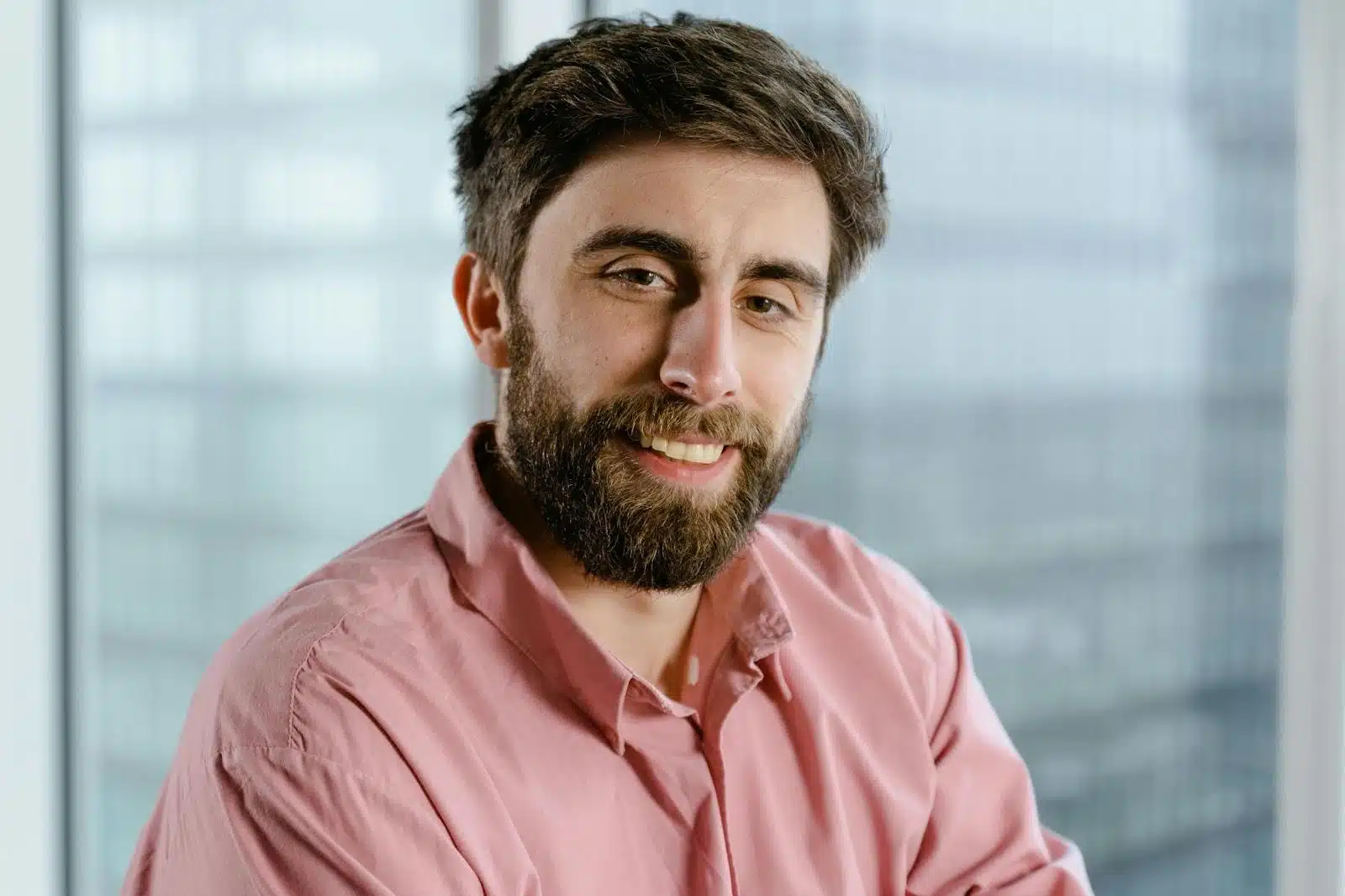
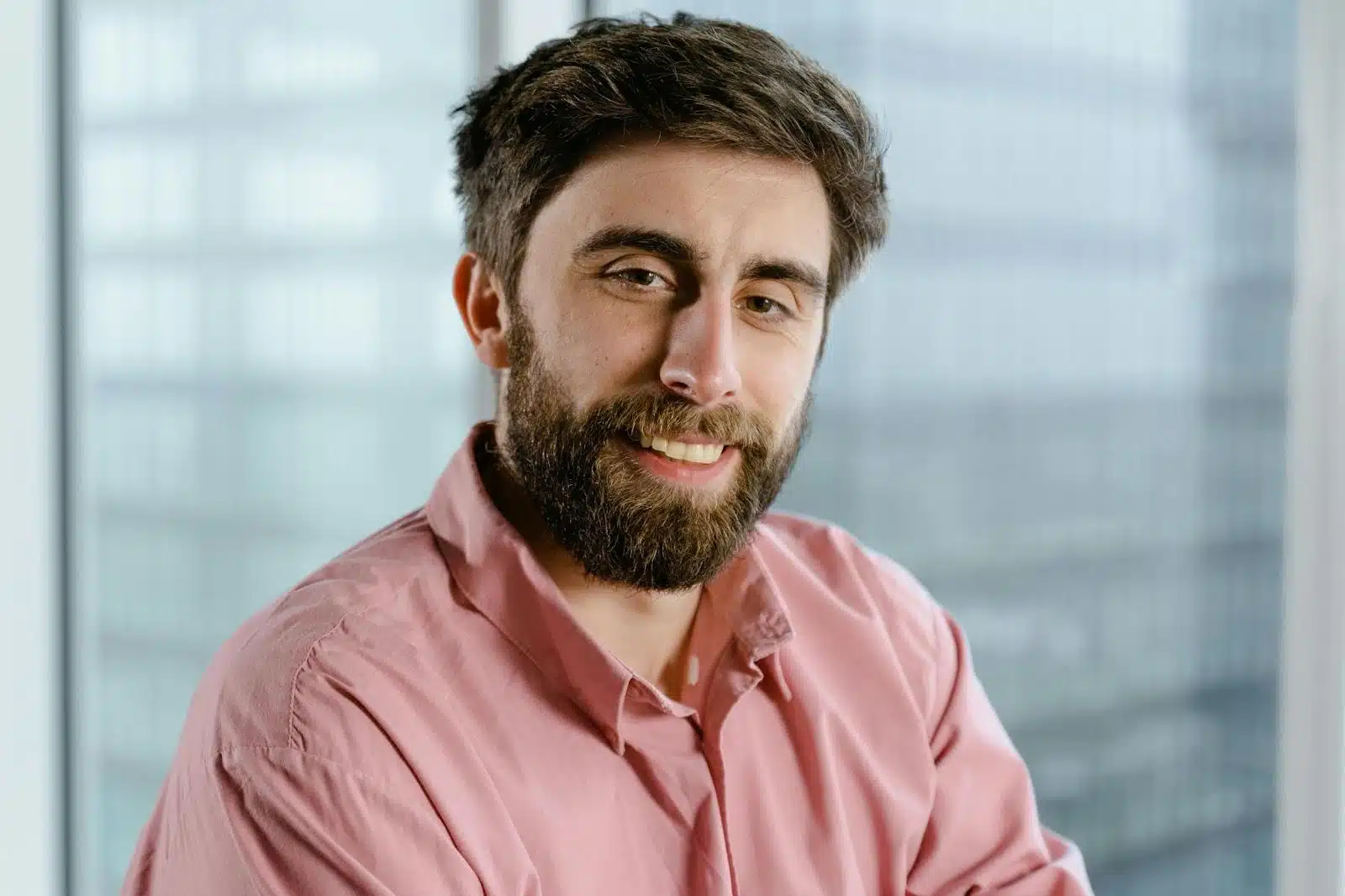
Credits to: portraitpal.ai
One thing I love is the variety of styles. Whether you’re going for a polished, suit-and-tie professional look or something more laid-back. The subtle smiles it adds feel friendly but not over-the-top, and the color tones are muted enough to scream “professional” without being boring.
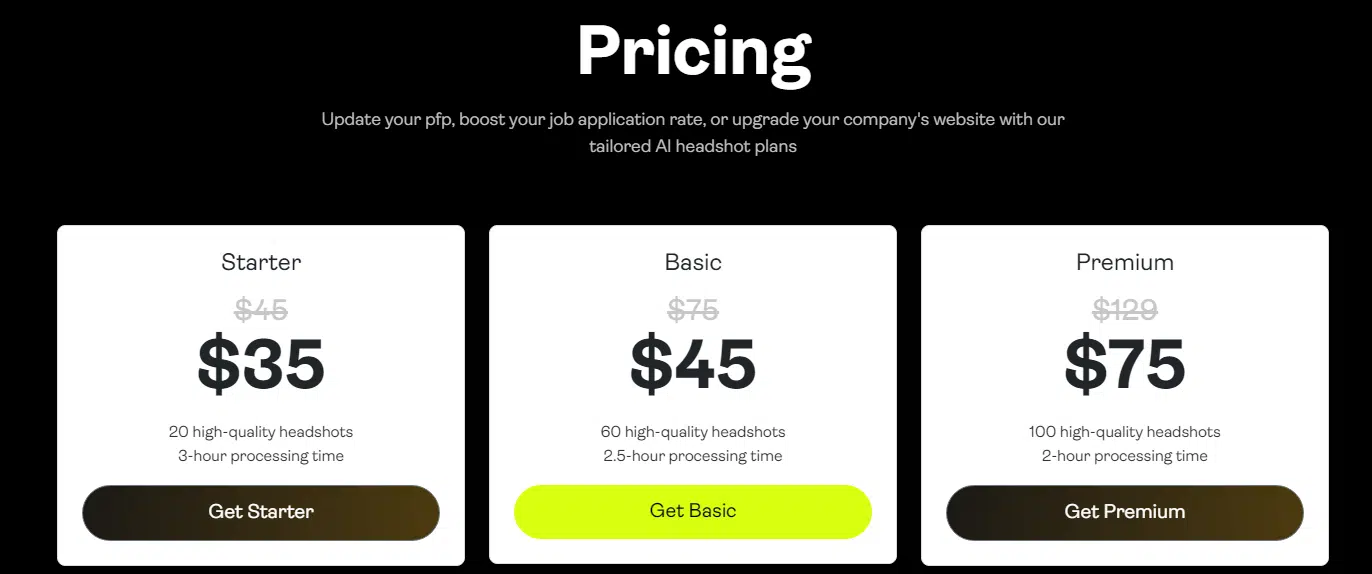
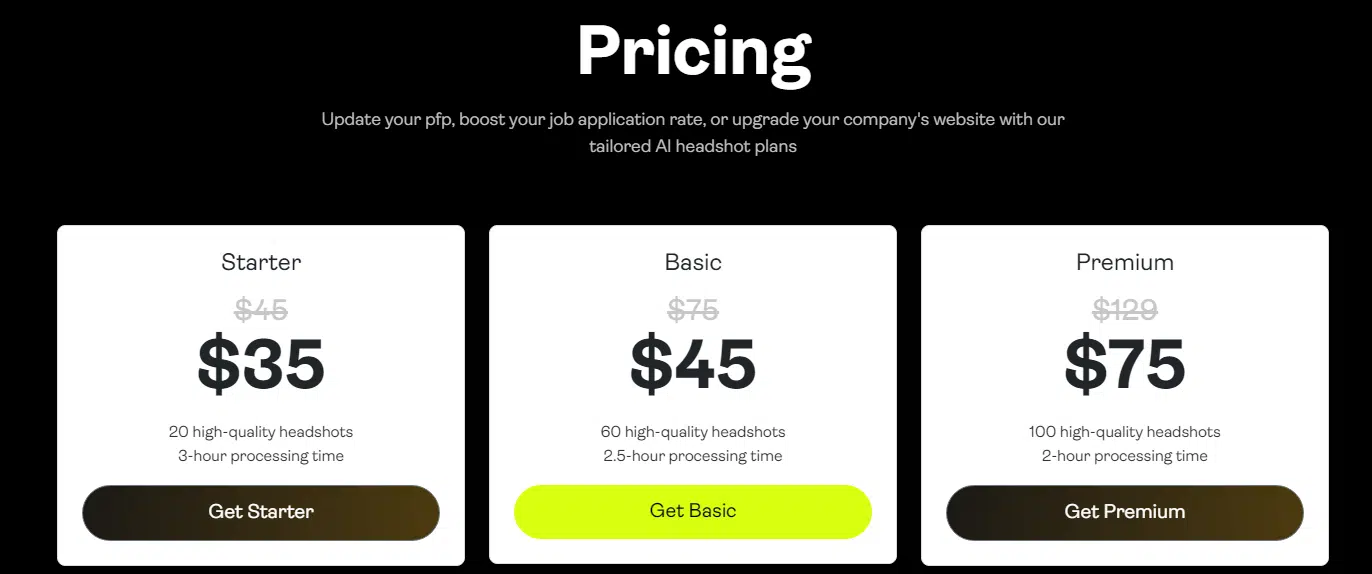
It’s no surprise that Portrait Pal is one of the most popular AI headshot generators out there. When you sign up, you’ll choose from three pricing tiers, all reasonably priced. Each tier gives you a little more—faster processing, more headshots, more outfits, and more backgrounds.
After you upload your photos (the more variety, the better), the tool uses stable diffusion AI and some clever fine-tuning to whip up your headshots. In a couple of hours—or just 30 minutes if you spring for the top tier—you’ll have a collection of images ready to go.
#2 PixelPose
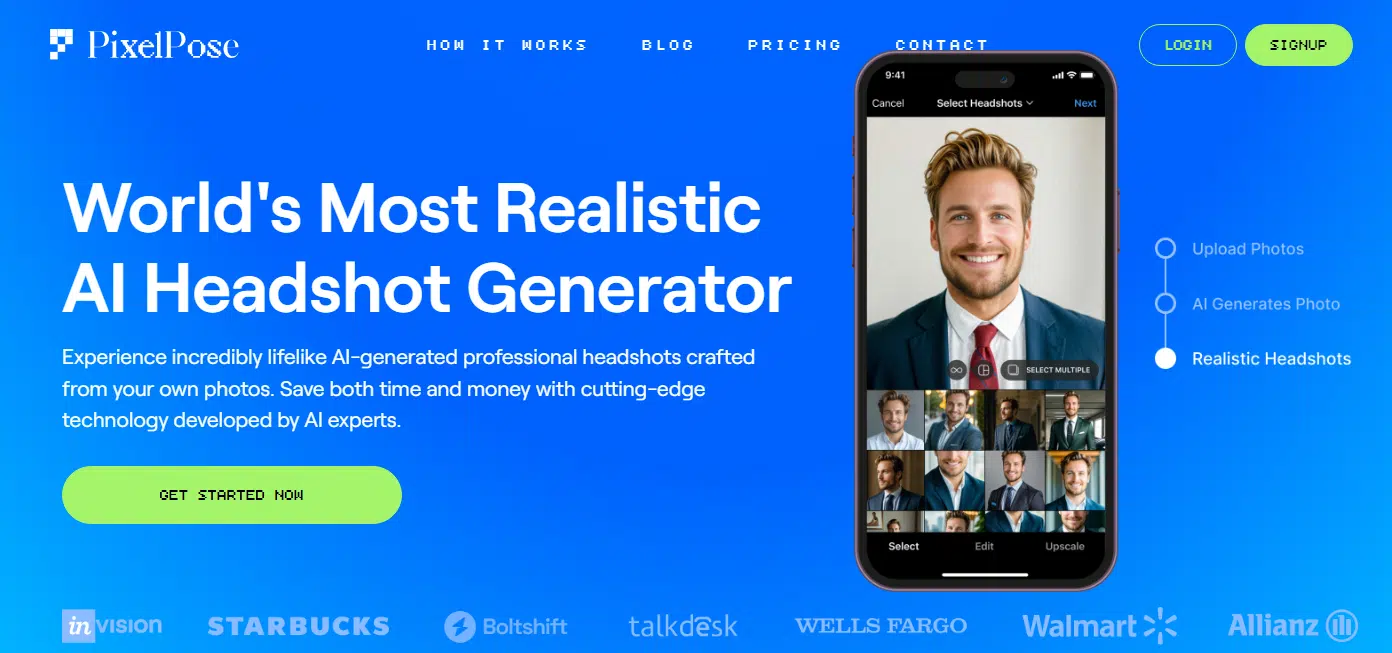
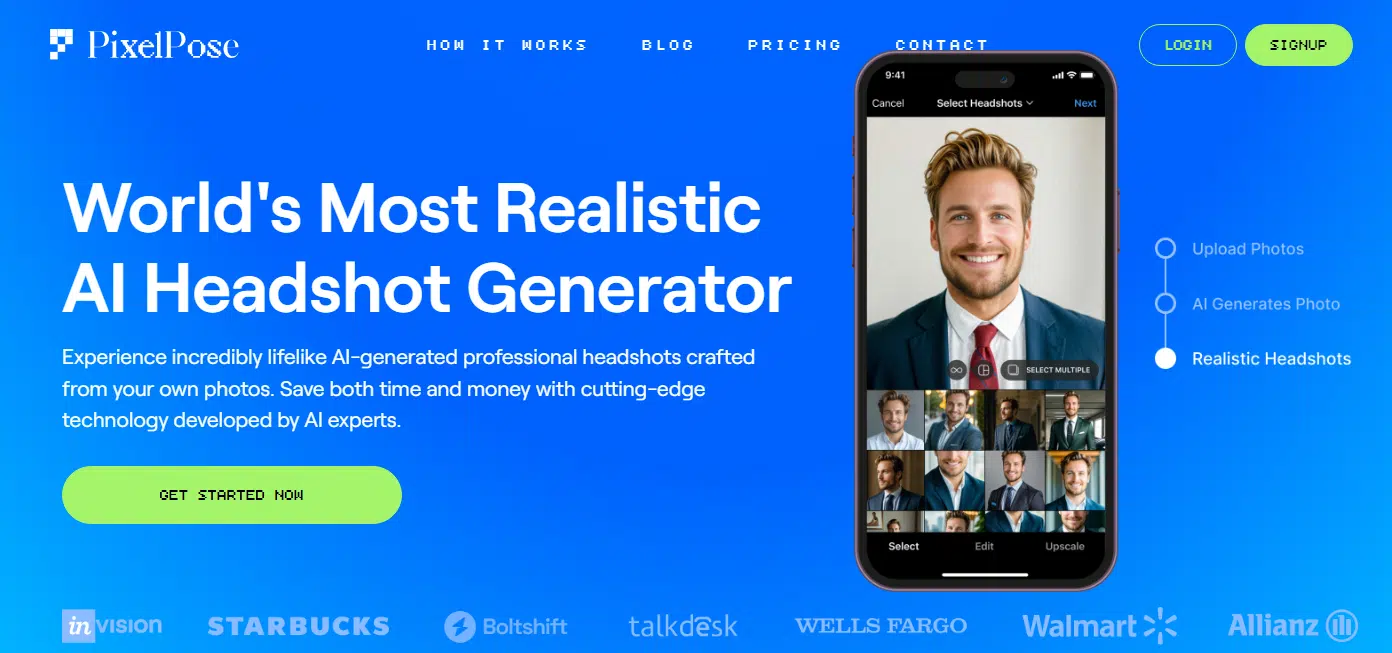
PixelPose is up next, and it is very impressive. The quality and realism of the headshots are seriously next-level. What makes it even cooler? It’s a brand-new service, so it’s rocking the latest and greatest AI tech out there.
The AI does an amazing job of capturing your look. Some tools might get a few angles or features right, but PixelPose nails it across the board. Every photo feels consistent, like it actually knows what you’d look like in different settings.
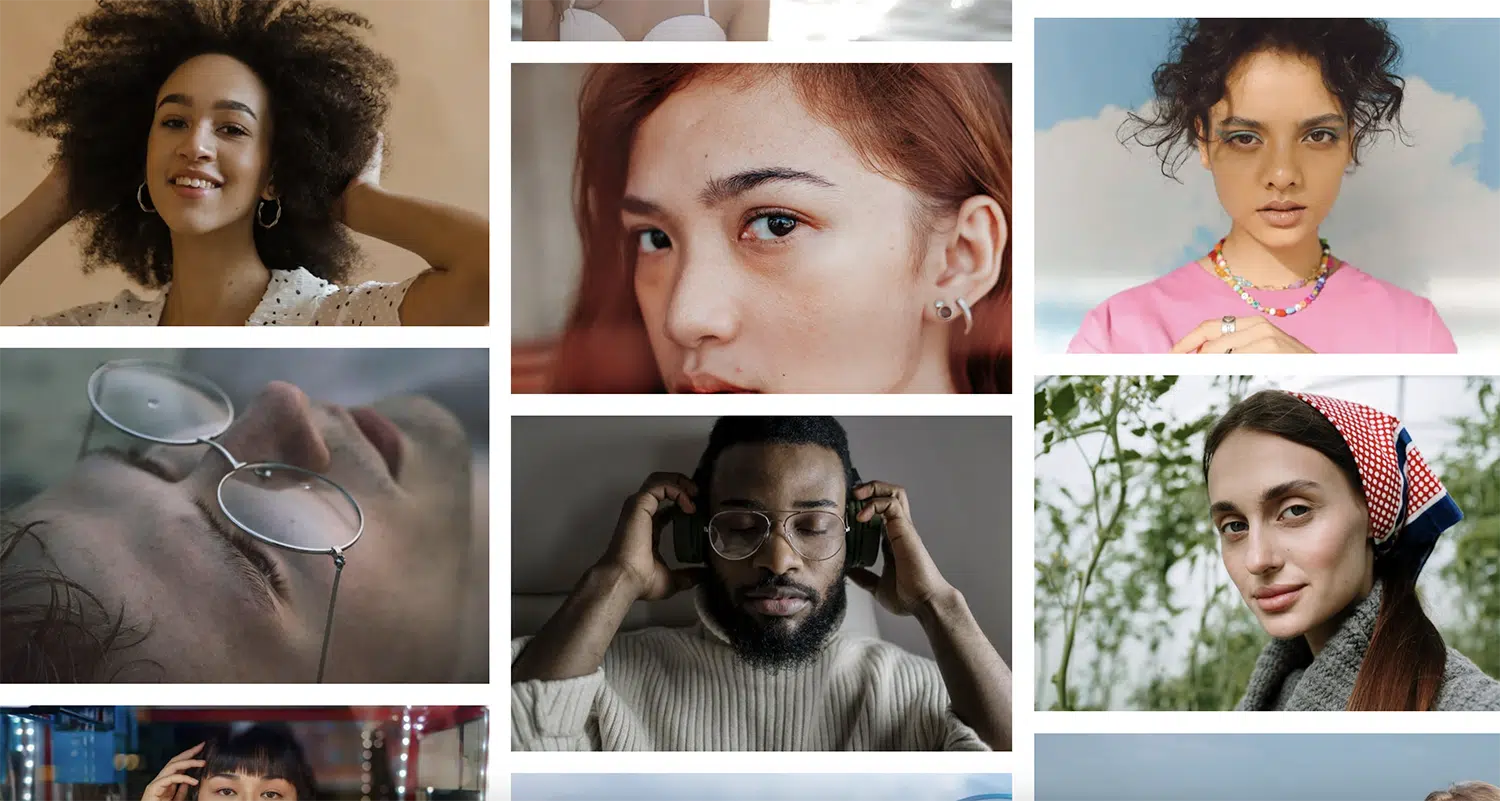
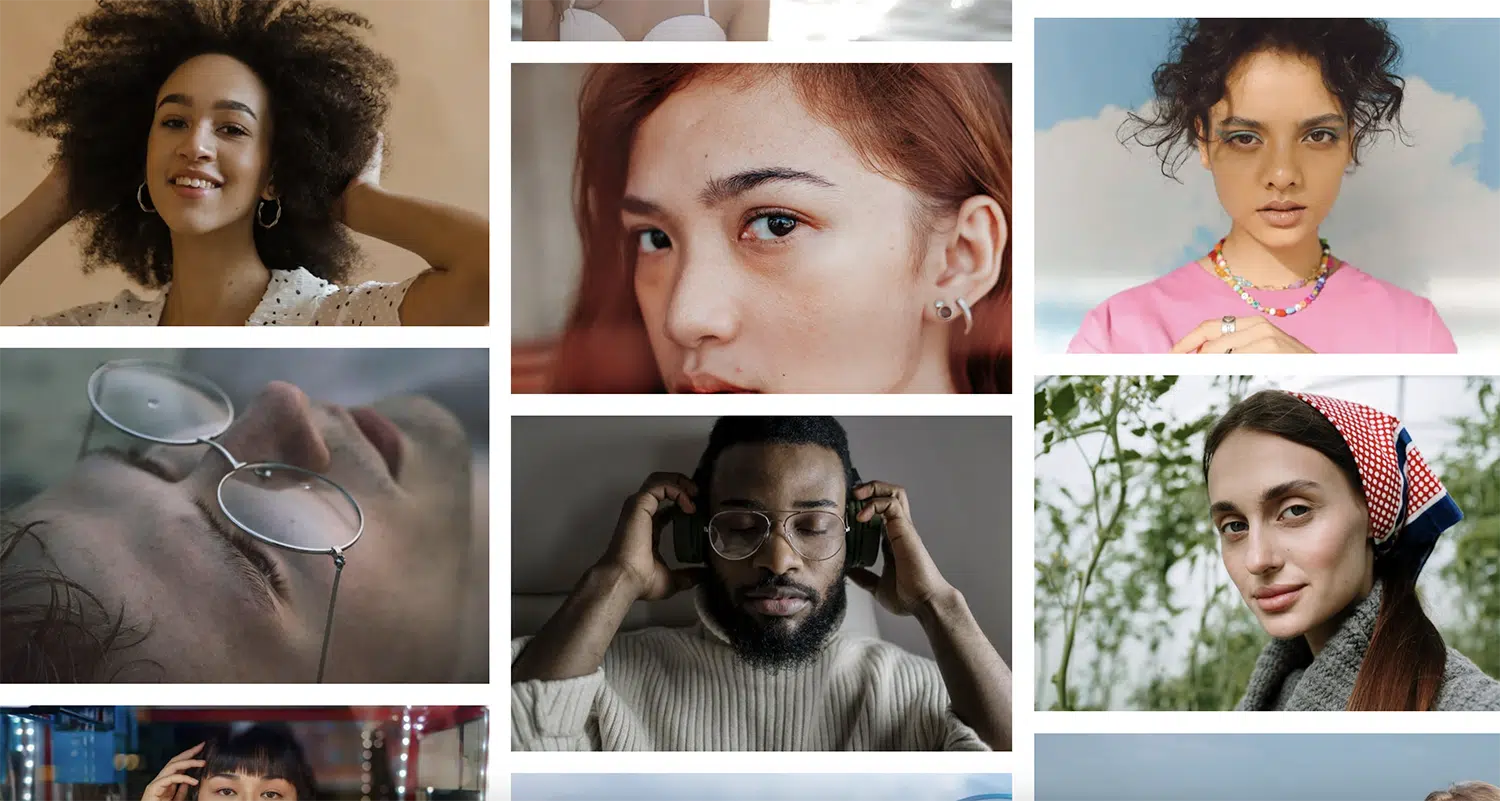
Credits to: PixelPose.ai
The headshots it spits out are super polished. Most of the poses look sharp, with clean suits and no weird AI glitches to throw you off.
Hands? Either hidden or totally natural. Clothes? Smooth and layered perfectly, without any of those strange errors you sometimes see with AI.
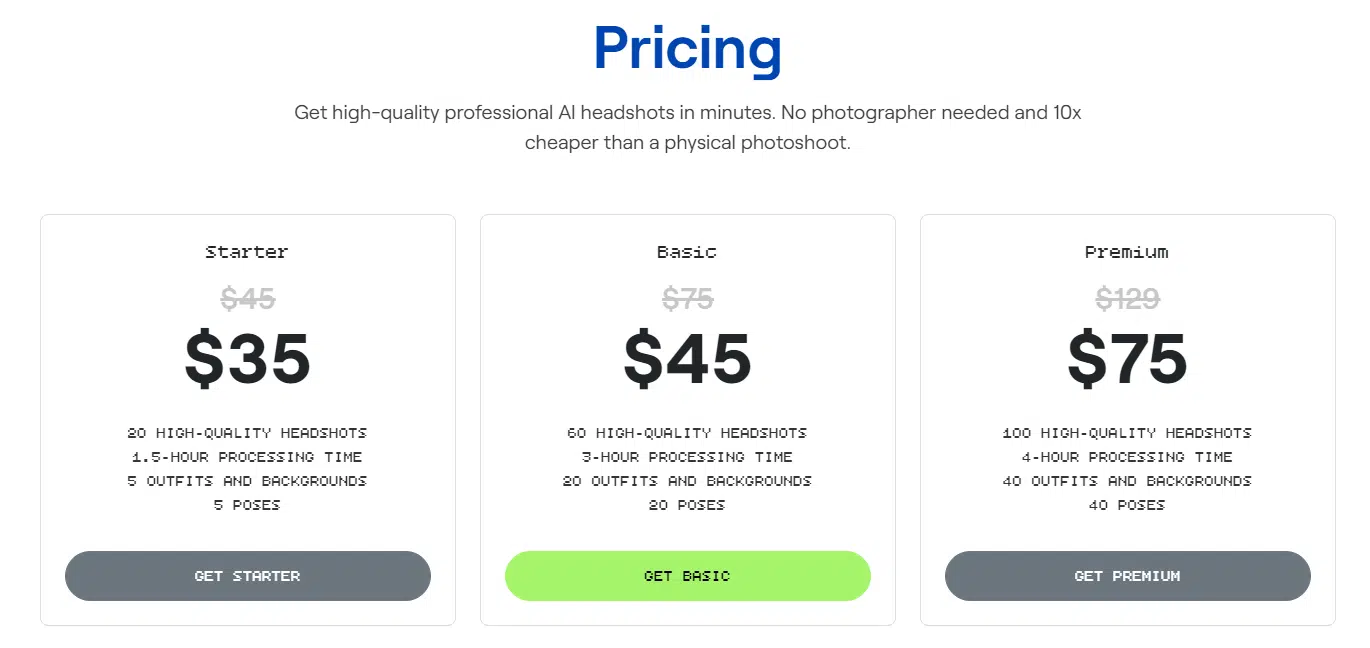
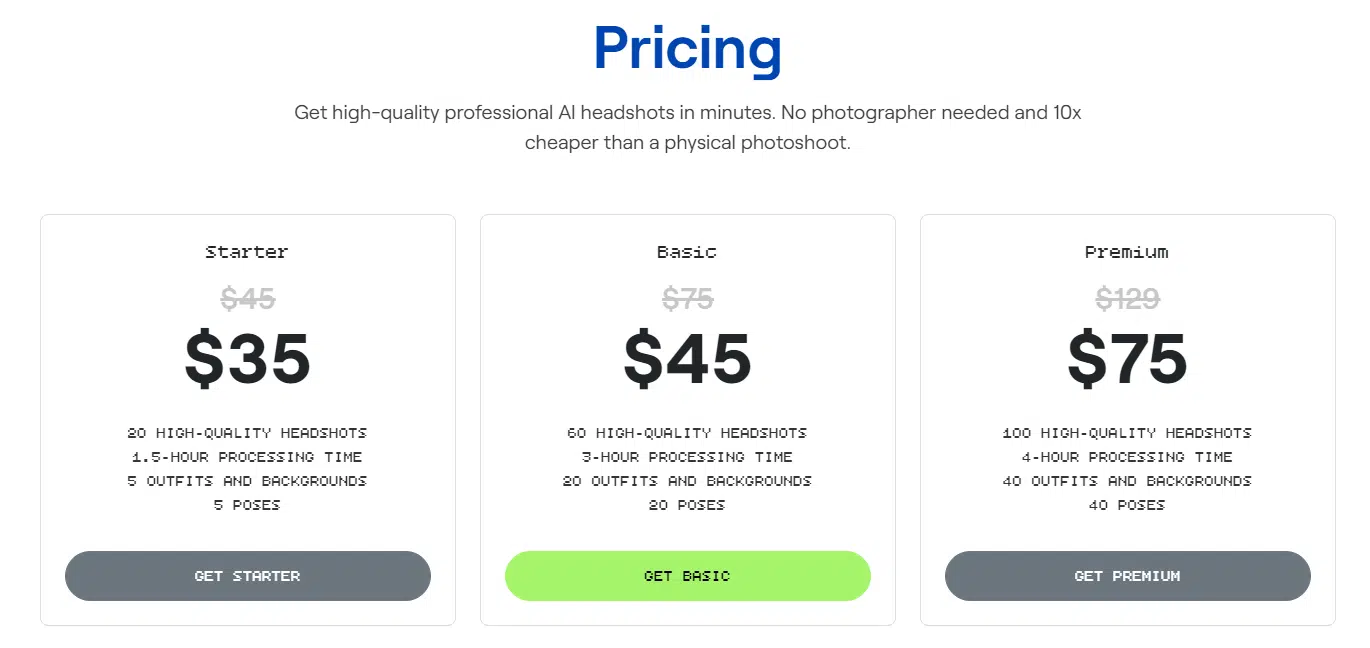
You’ve got three pricing tiers to pick from, and the higher you go, the more HD images and styles you’ll get. There isn’t a ton of customization, but, that makes it so easy to use. Just upload your pics, let the AI do its thing, and boom—you’ve got a batch of headshots ready to go.
Pick your favorites, and the rest get saved in case you need them later.
#3 Remini
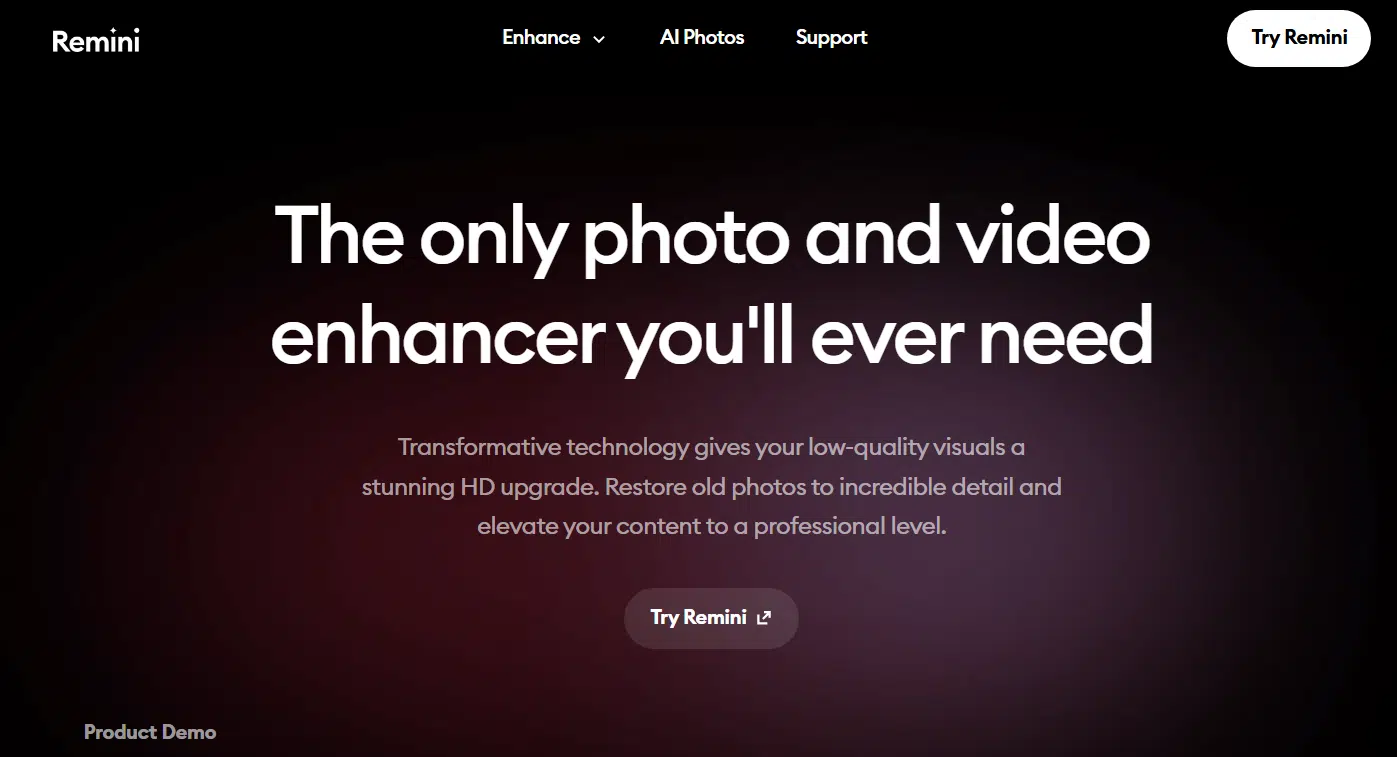
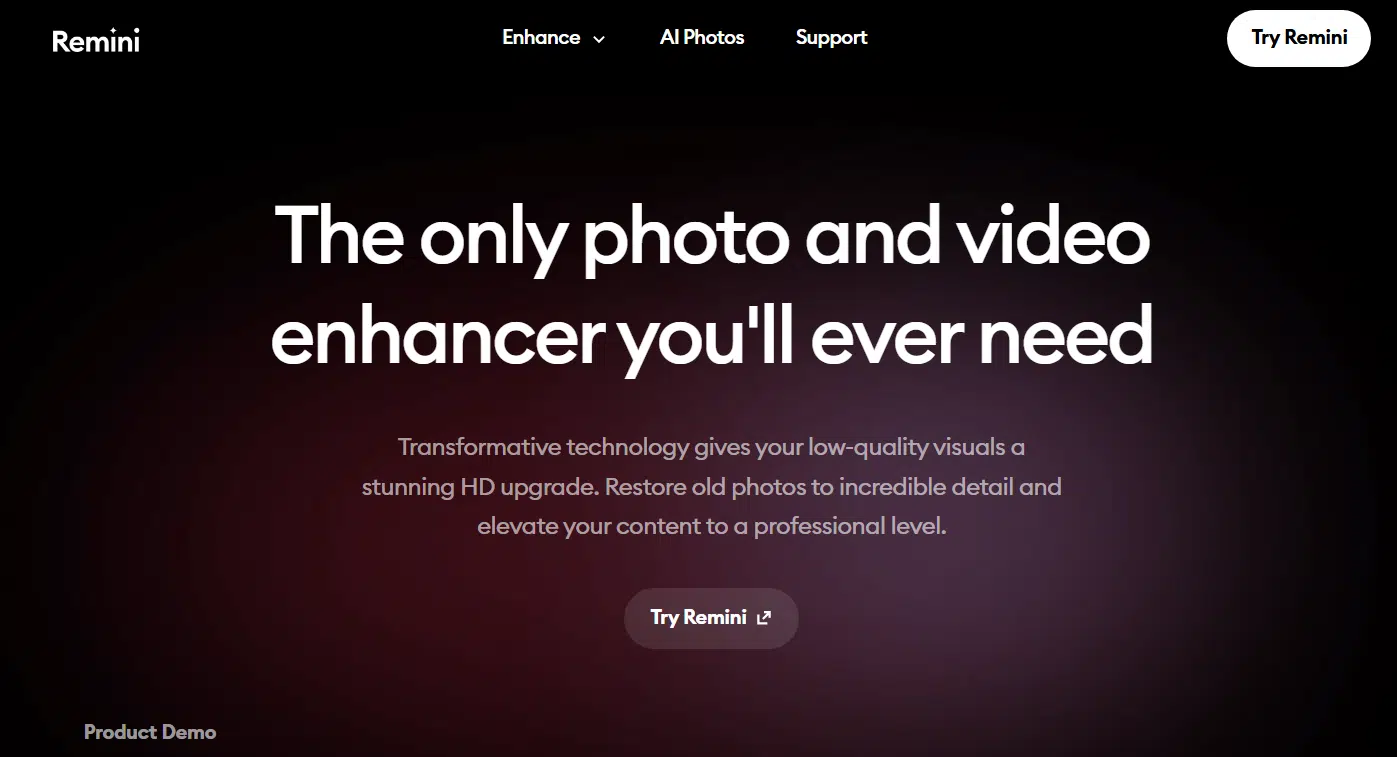
Let’s talk about Remini, another headshot generator that’s got people buzzing. The photos it produces are almost as realistic as what you’d get with Portrait Pal, though it doesn’t quite hit the same level when it comes to capturing your exact likeness. Still, these images are pretty convincing—you could easily mistake them for actual photos.
That said, there are a few quirks that keep Remini from taking the top spot. For one, like most AI tools, it still struggles with hands.
Another thing? The images have this shiny, almost “post-workout glow” going on. It’s not super obvious in the brighter images, but once you spot it, you can’t unsee it.
Style-wise, the photos lean more relaxed. Think loose collars, turtlenecks, and laid-back poses. This vibe works great for casual or creative headshots, but if you’re aiming for something ultra-professional, these might not quite hit the mark.
Remini does give you some cool customization options, though. You can pick models with specific poses as a starting point, which might help you create a more polished, professional look. Plus, the colors and contrast are solid, and if you stick with a simple background, the results feel even sharper and more put-together.
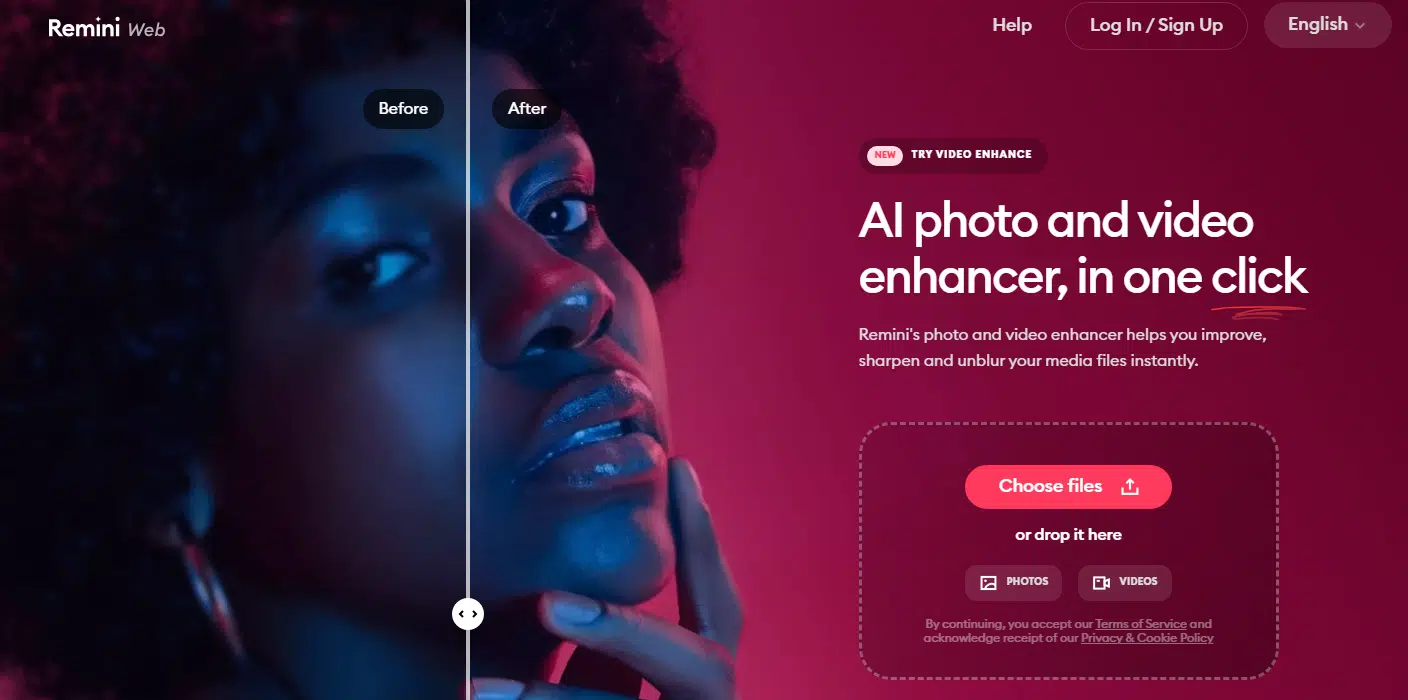
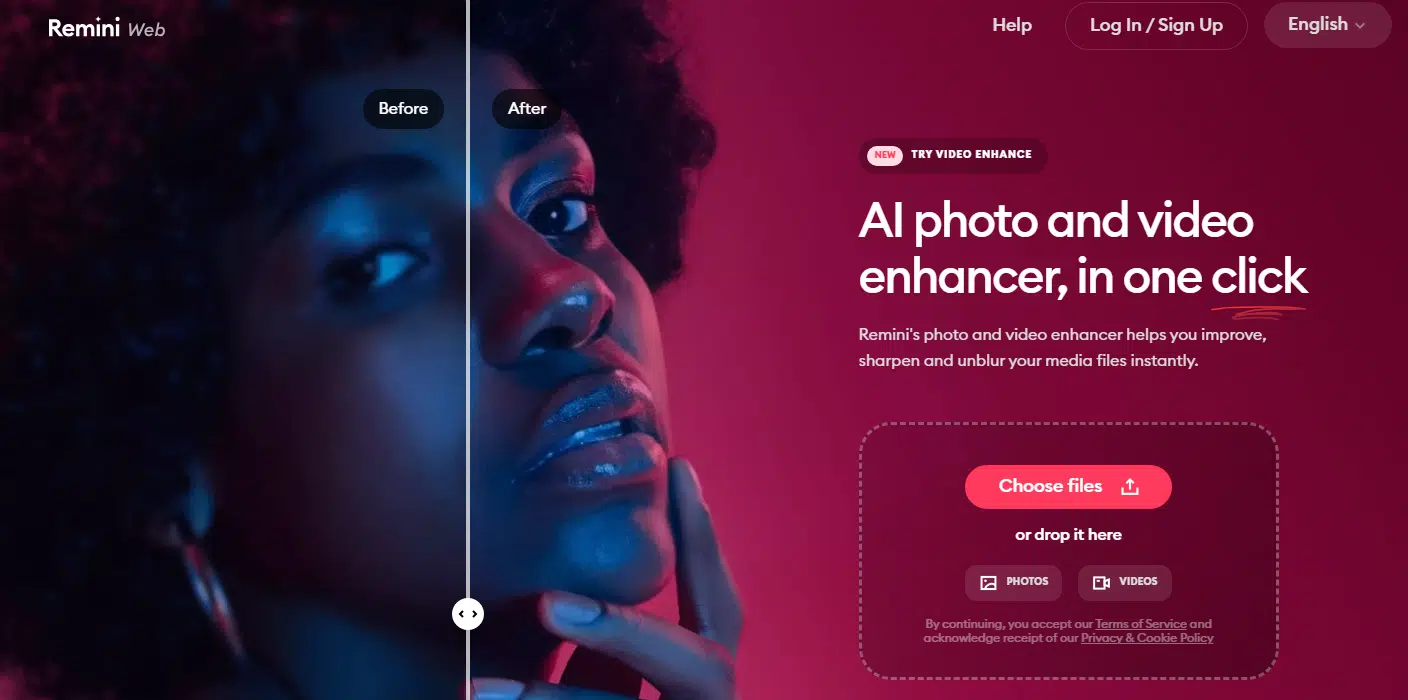
Beyond headshots, Remini can also enhance existing photos or create AI images in all kinds of styles. You might come for the headshots but end up experimenting with its other features, too. Just keep in mind that while photo enhancement works on the web, the AI image generator is only available through their mobile app.
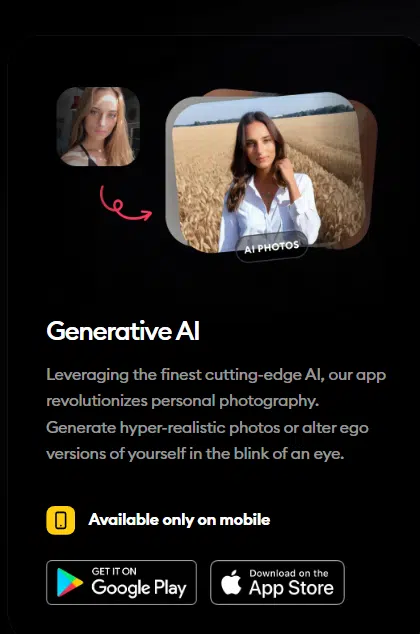
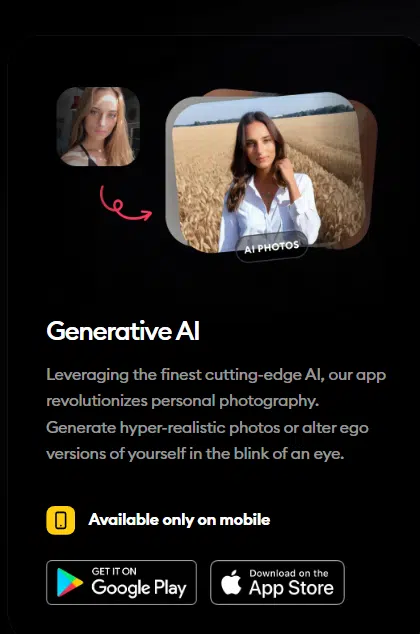
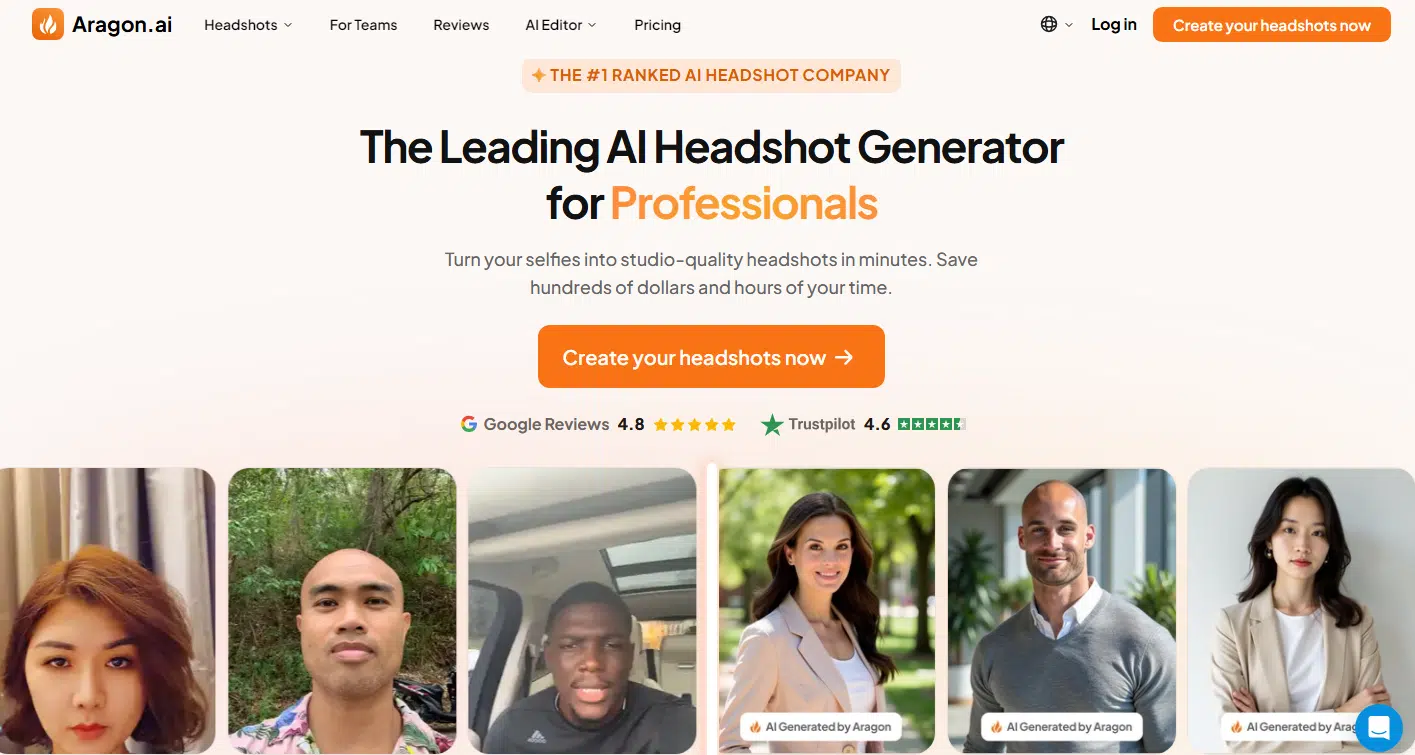
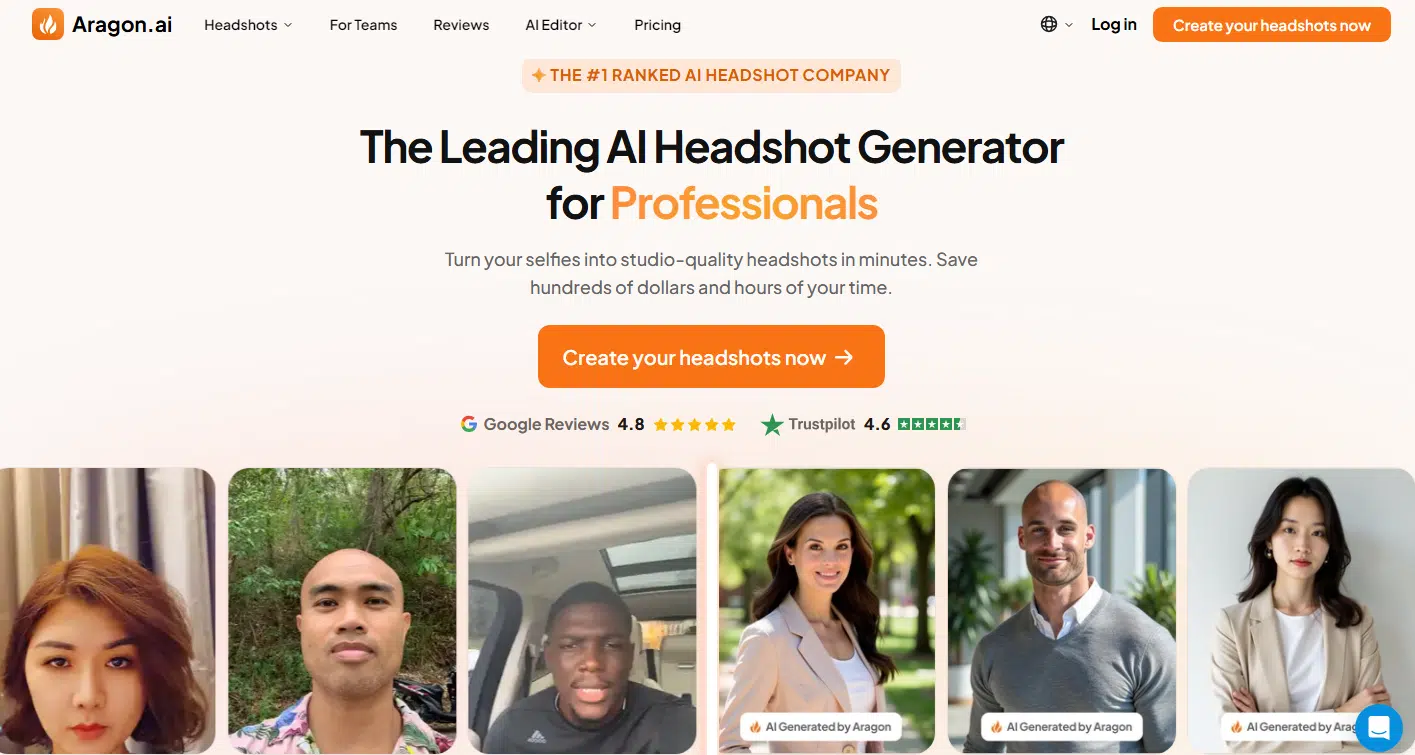
Aragon.ai left a solid first impression, earning its spot in fourth place. The headshots it churned out looked pretty realistic, though they leaned a little bright—almost like someone slapped a subtle filter on real photos. That said, the likeness was spot-on, and the results definitely felt professional.
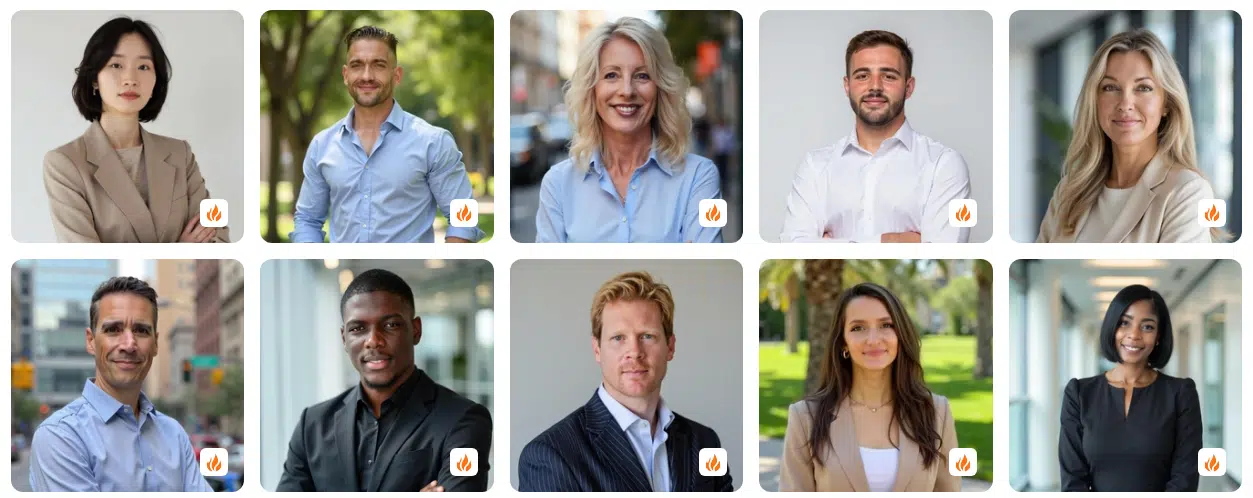
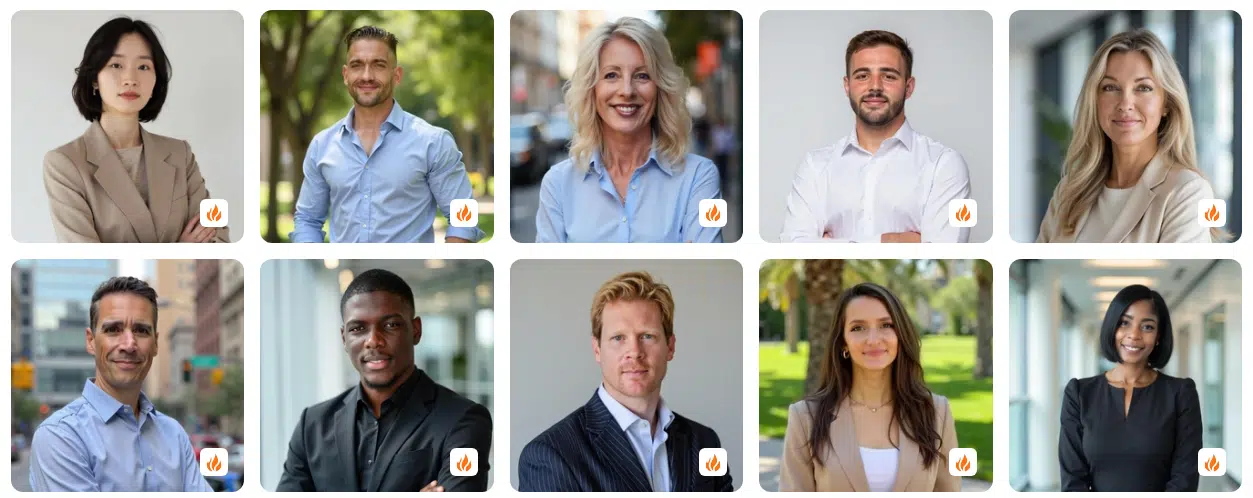
Credits to: Aragon.ai
What really stood out to me was the variety. A lot of AI generators stick with one expression and just change the angles or backgrounds, but Aragon.ai mixed things up nicely. The photos ranged from slight, friendly smiles to full-on grins, with plenty of outfit options. You get everything from formal suits and ties to more relaxed, casual looks.
Using Aragon.ai is ridiculously easy, too. It’s not just for professionals—it’s got presets tailored for students, teachers, doctors, and other careers. Plus, there’s an AI editor you can use to touch up or tweak photos you already have.
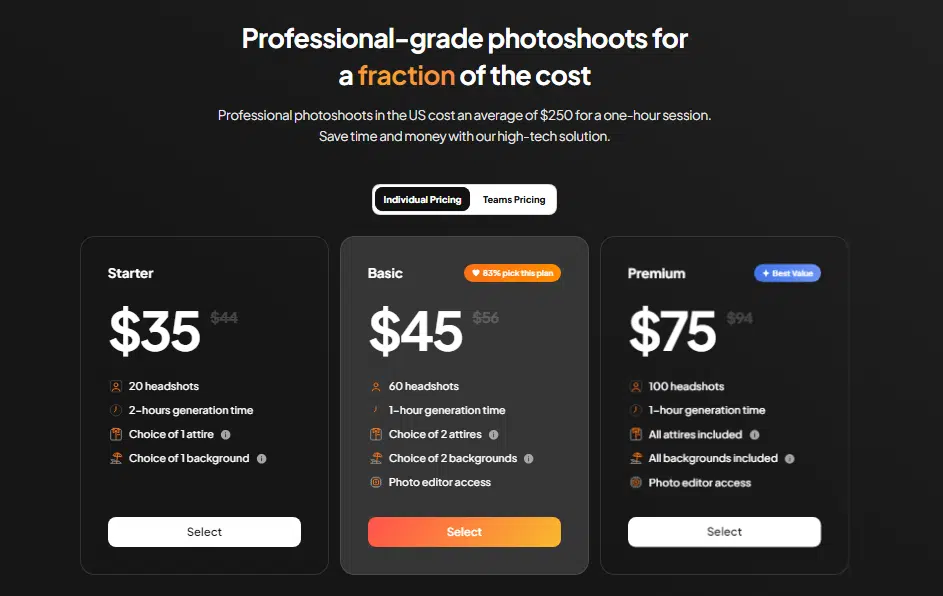
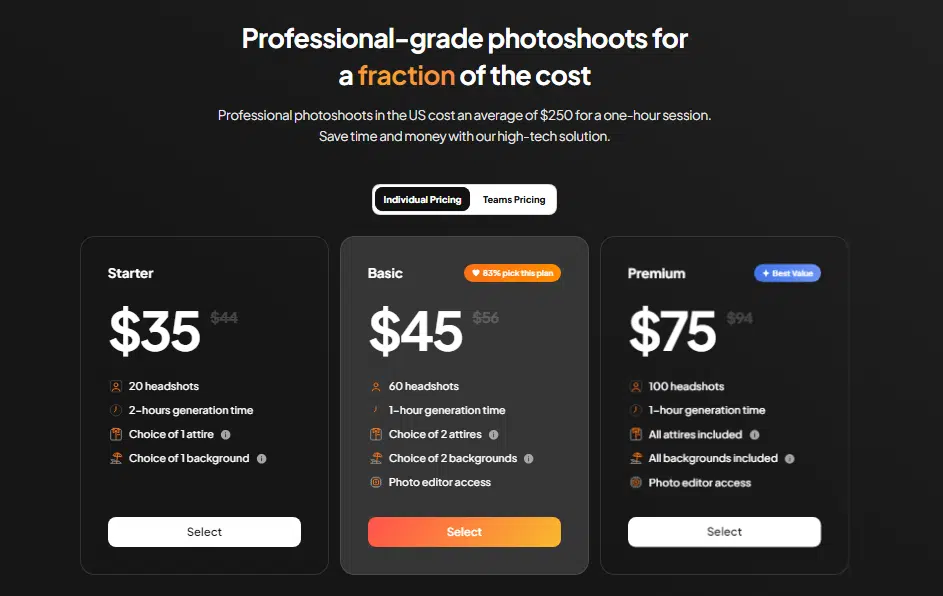
Pricing-wise, Aragon.ai follows the trend most AI headshot generators set: three tiers to choose from. The higher the tier, the more poses, backgrounds, and options you get.
One cool feature? There’s even a team plan, which is great if you want a batch of consistent headshots for a company’s “about us” page.
#5 TheMultiverse
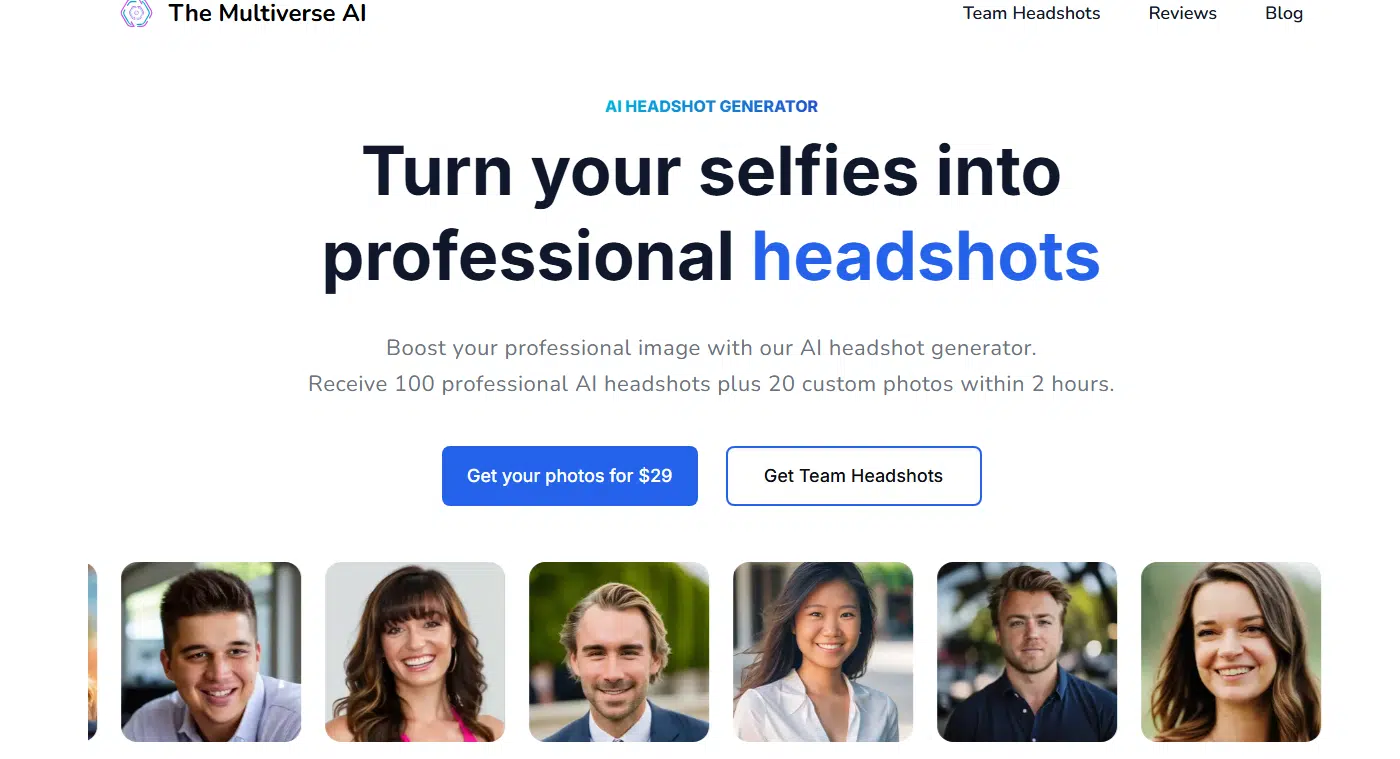
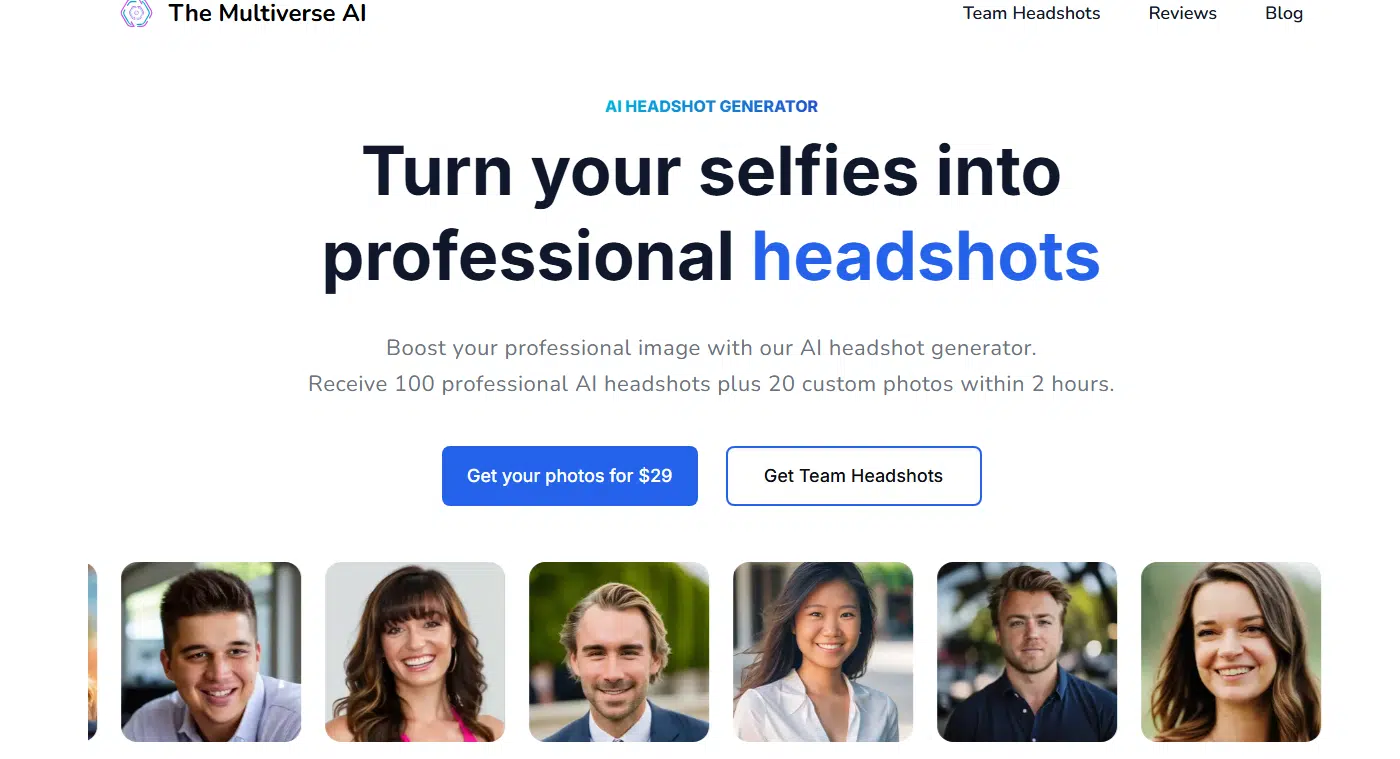
The Multiverse AI takes fifth place on this list, and it stands out for one thing: its photos have this warm, sunny vibe. If you’re into headshots that look like they were snapped on a perfect summer afternoon, this one’s for you.
The images it produces are undeniably solid, but The Multiverse AI does stick to a single look. You’ll get variations in angles, backgrounds, and poses, but the overall vibe doesn’t change much. Most of the photos lean toward lighter clothing—think tans and whites—with a few navy blues mixed in. It’s consistent, but that can be both a pro and a con.
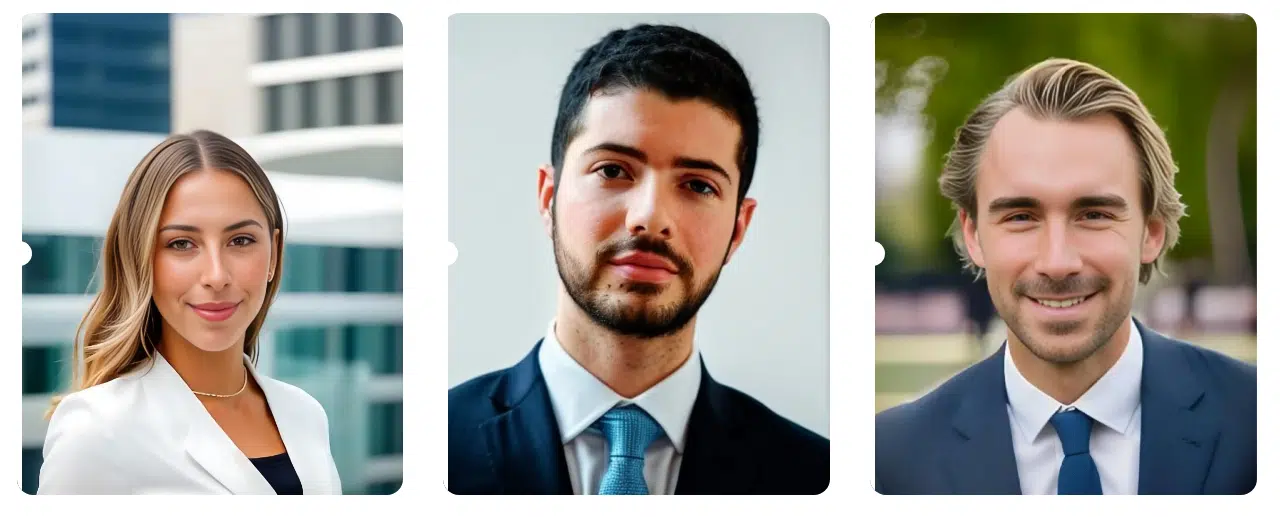
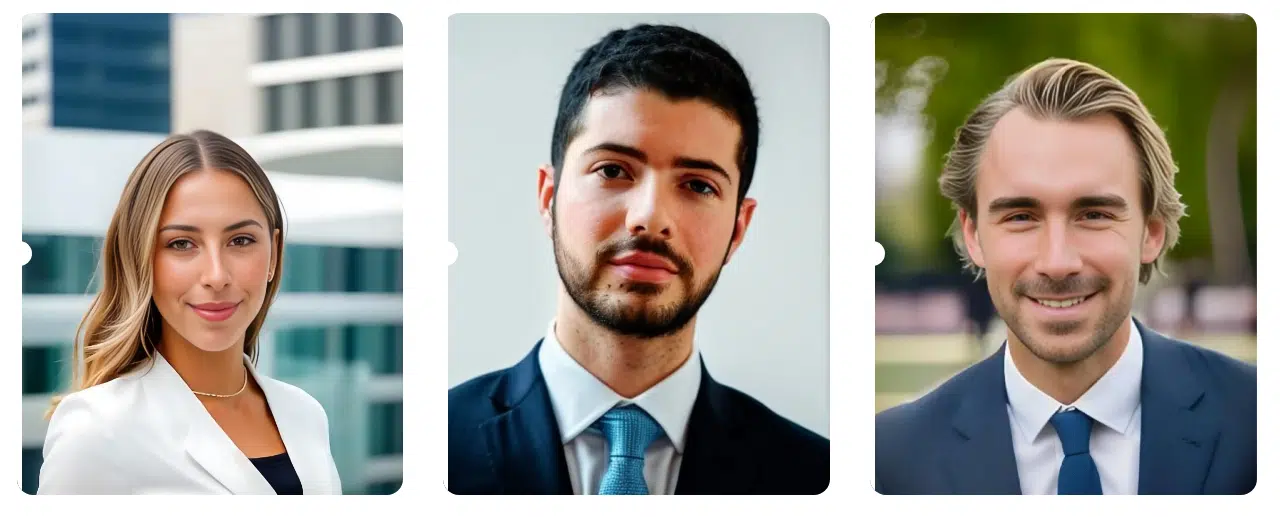
Credits to: The Multiverse AI
Here’s the thing, though: seeing multiple versions of yourself with nearly identical expressions can feel a little… strange.
Still, the tool knows what it’s good at and sticks to it. If you’re happy with the style, you’ll end up with plenty of headshots to work with. Just don’t look too closely at the fingers in some of the photos—it’s better not to ask questions.
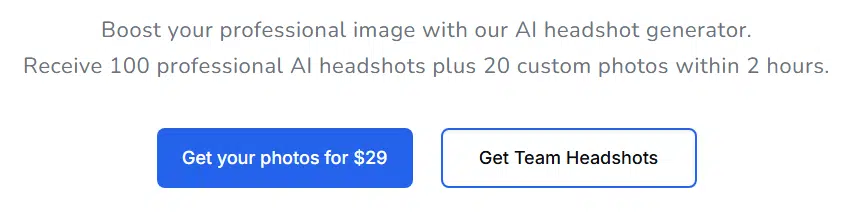
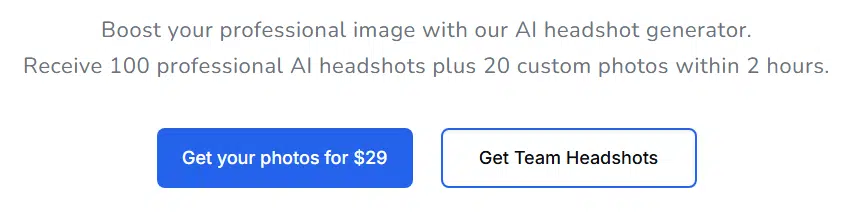
The Multiverse AI keeps it simple with one price point. After you pay, you’ll get an email link where you can upload 12 to 20 images. A few hours later, another email arrives with a fresh batch of headshots ready for you.
Customization isn’t its strong suit, but it makes up for that by offering 100 photos upfront. Plus, you can request 20 custom images by submitting a specific prompt—so if you’ve ever wanted to see yourself in a certain role or outfit, here’s your chance. They even have a team option if your whole organization needs consistent headshots.
#6. InstaHeadshots
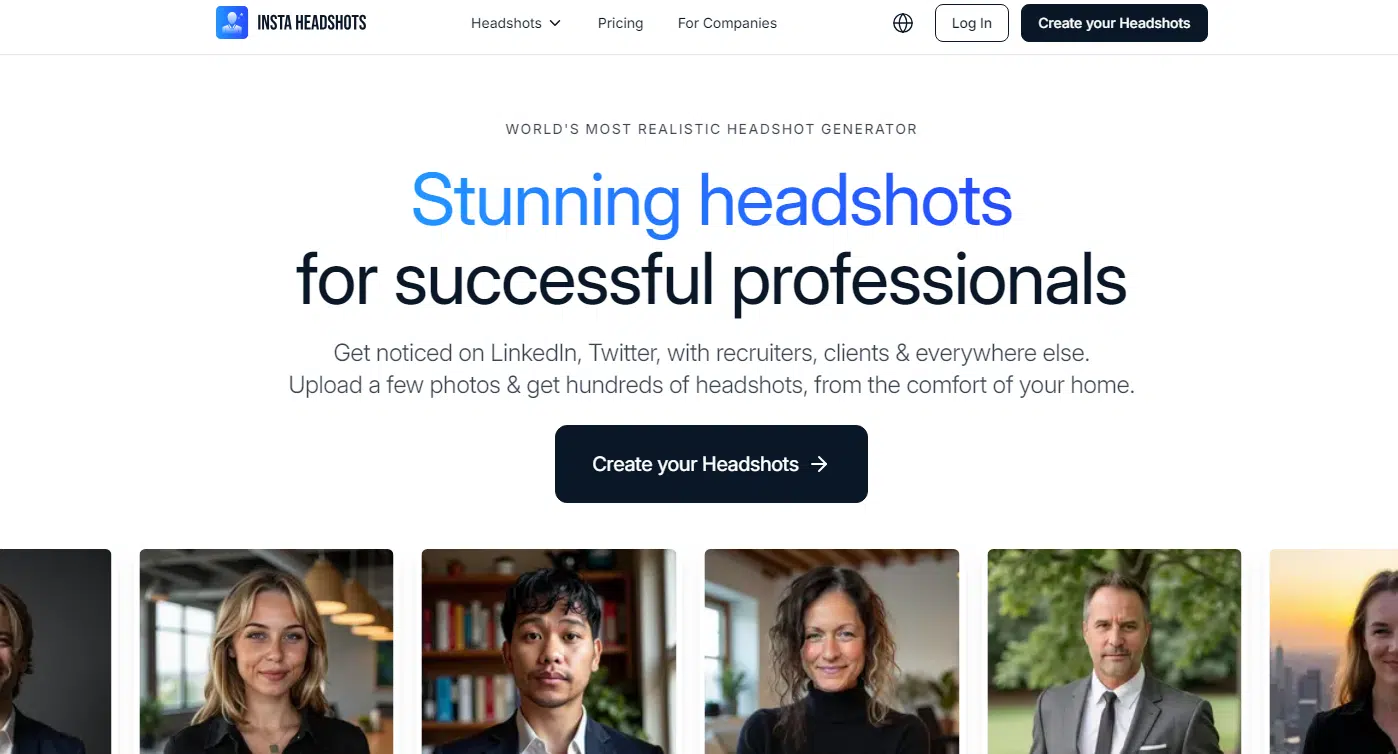
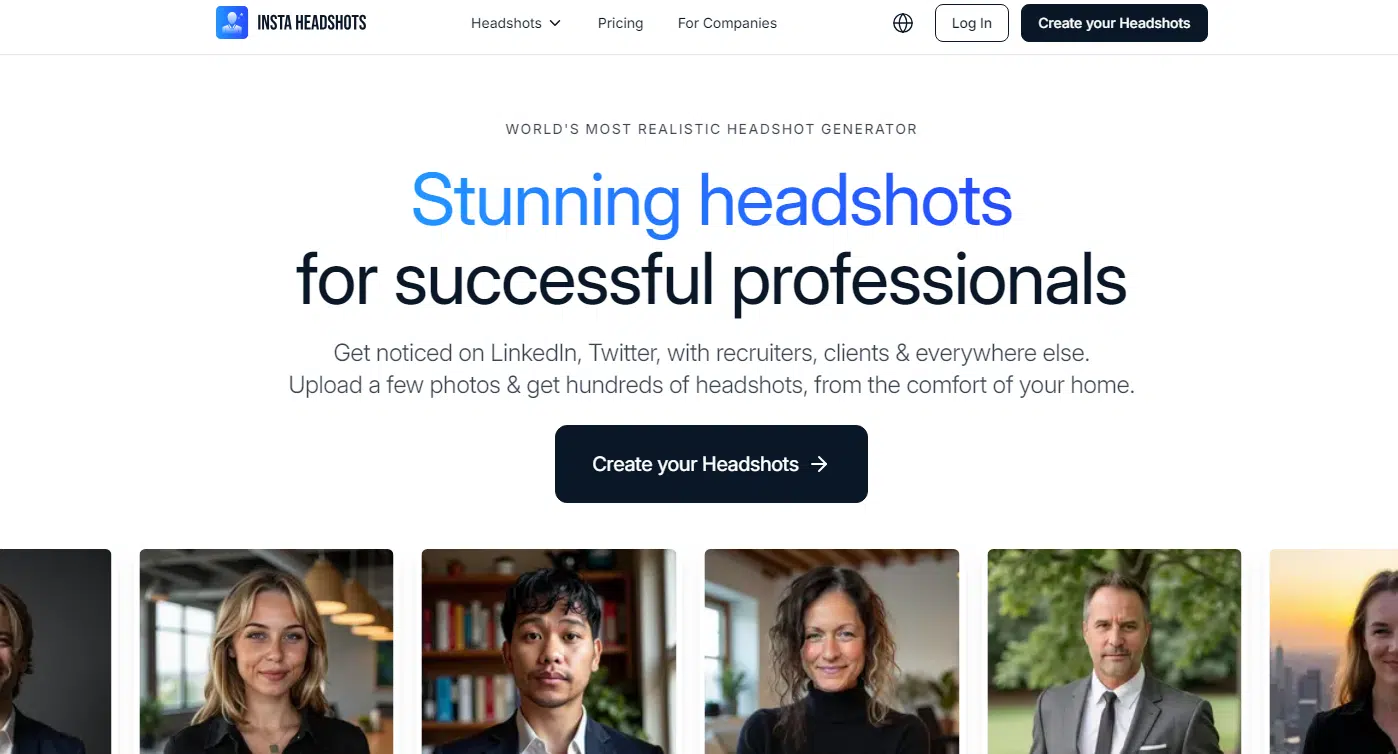
InstaHeadshots lands right in the middle of the list, and it’s got a cool vibe going on. While Aragon.ai and The Multiverse AI lean warm and sunny, InstaHeadshots goes for cooler blues and greens. Some of the shots really pop, like the green suit with the high contrast, which definitely stands out.
The facial expressions? Eh, not much variety there.
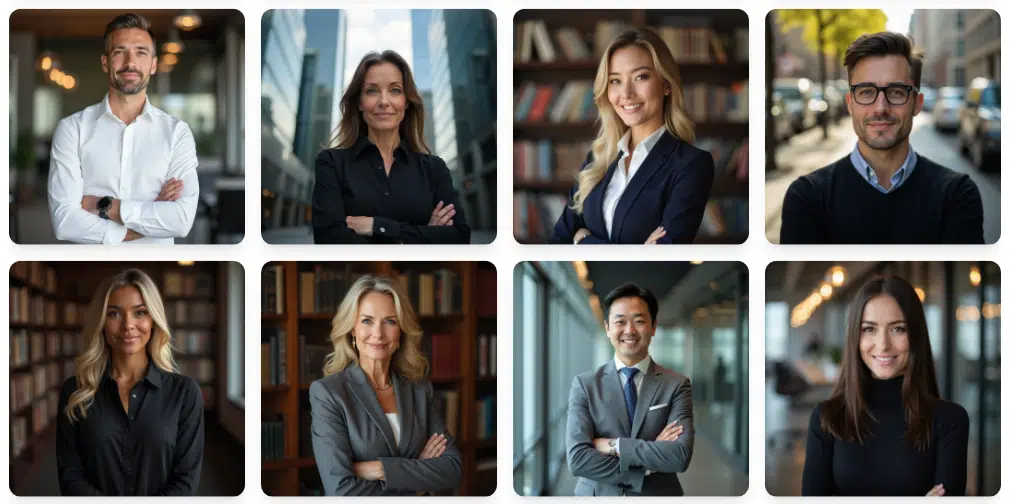
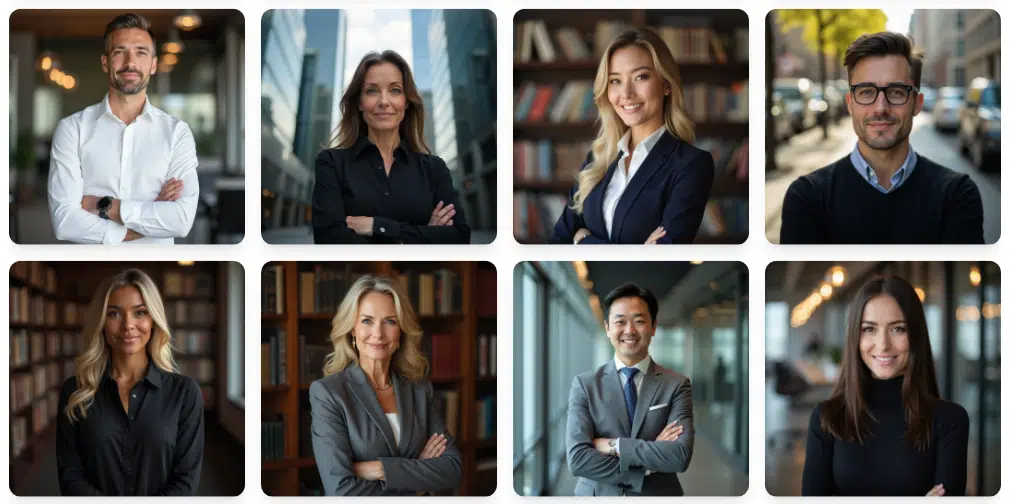
Credits to: InstaHeadshots
Most of the shots feel like the same look just from slightly different angles. It still kinda looks like the person, but not as spot-on as Portrait Pal or some of the other top contenders. In a few cases, the headshots start to look more like digital art than actual photos.
That could work if you’re into a more creative style, but it’s not the best if you’re aiming for something super professional.
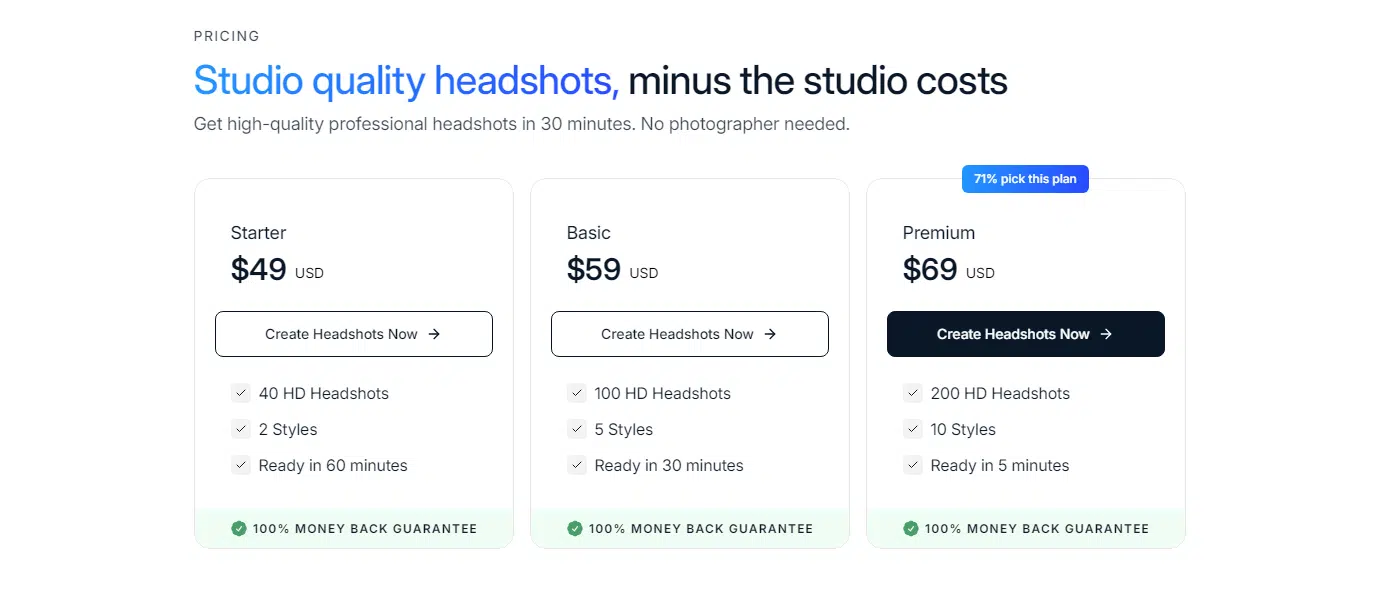
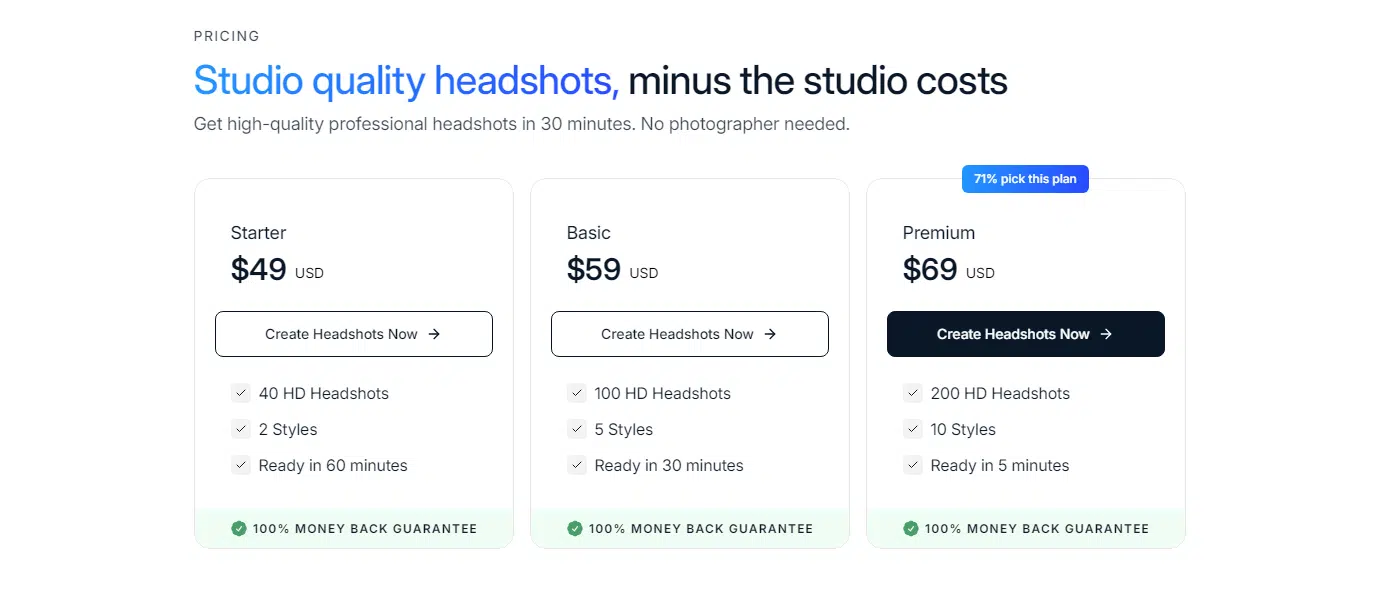
If you’ve tried other generators, InstaHeadshots will feel pretty familiar. You’ve got the usual three pricing tiers, and the more you pay, the more headshots and styles you get. The turnaround is quick—1 to 2 hours tops—and they’ve even got a team option if your company wants consistent headshots for everyone.
While it’s not the most consistent tool out there, InstaHeadshots definitely has its own vibe. Out of the pile of headshots it generates, you’re bound to find a few gems. If you like cooler tones and don’t mind a little artistic flair, give it a go!
#7 BetterPic


BetterPic brings something a little different to the table. Right off the bat, the photos feel kind of… utilitarian. Lots of grays and blues, giving off a super serious vibe. Honestly, the third shot even looks like it could belong to a Bond villain—very “dark and mysterious.”
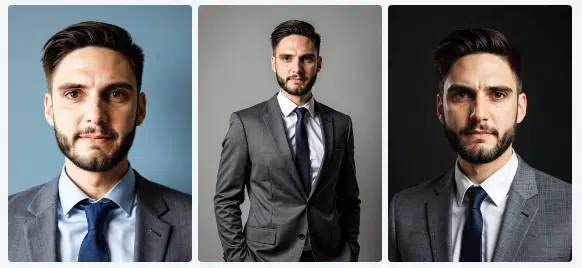
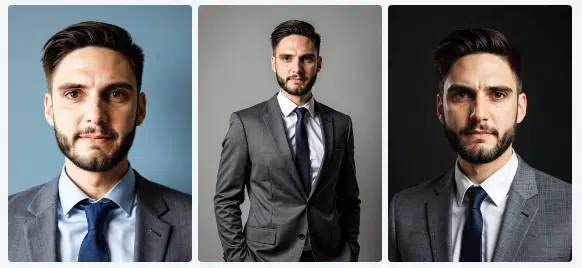
Credits to: BetterPic
The overall quality is decent, but not perfect. Some of the faces come out a little too red, and there’s this shiny, unnatural look in a few shots. That said, there are definitely some winners in the mix.
The Bond villain pic? Spot on. The second one? Pretty solid too.
What makes BetterPic stand out is the customization. Their Studio AI lets you tweak your headshots after the initial photos are generated. You can mess with everything—clothes, backgrounds, even hairstyles. Just pick your base photos, choose your outfits and styles, and go wild with the edits.
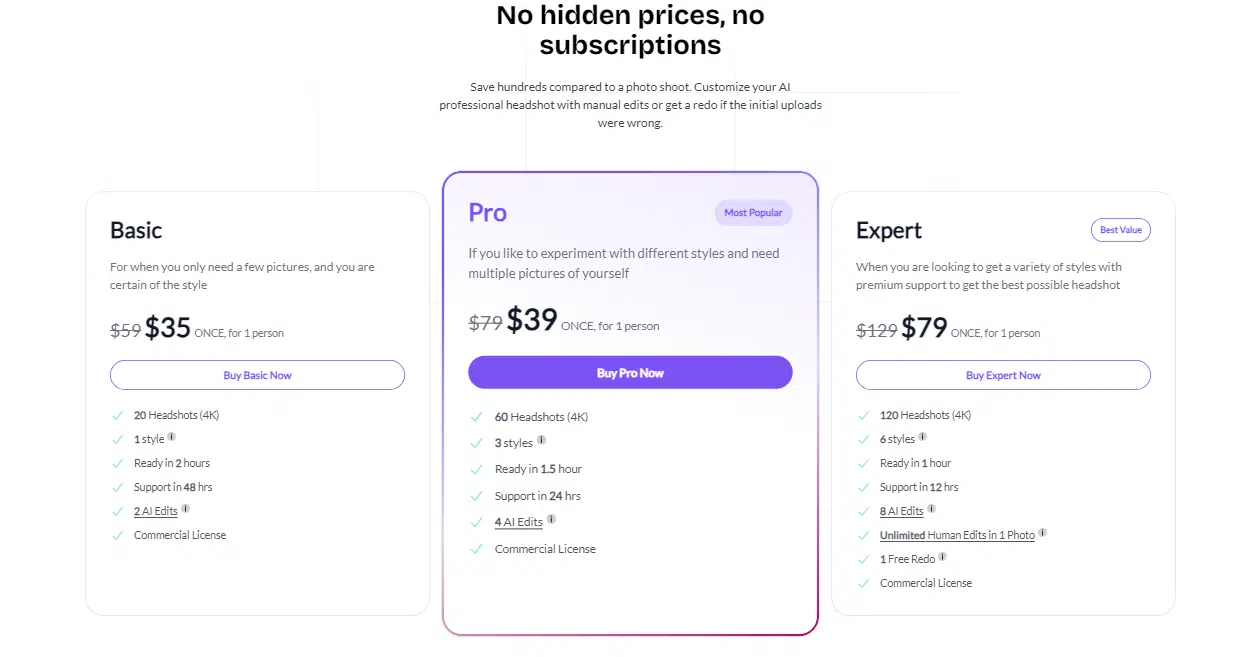
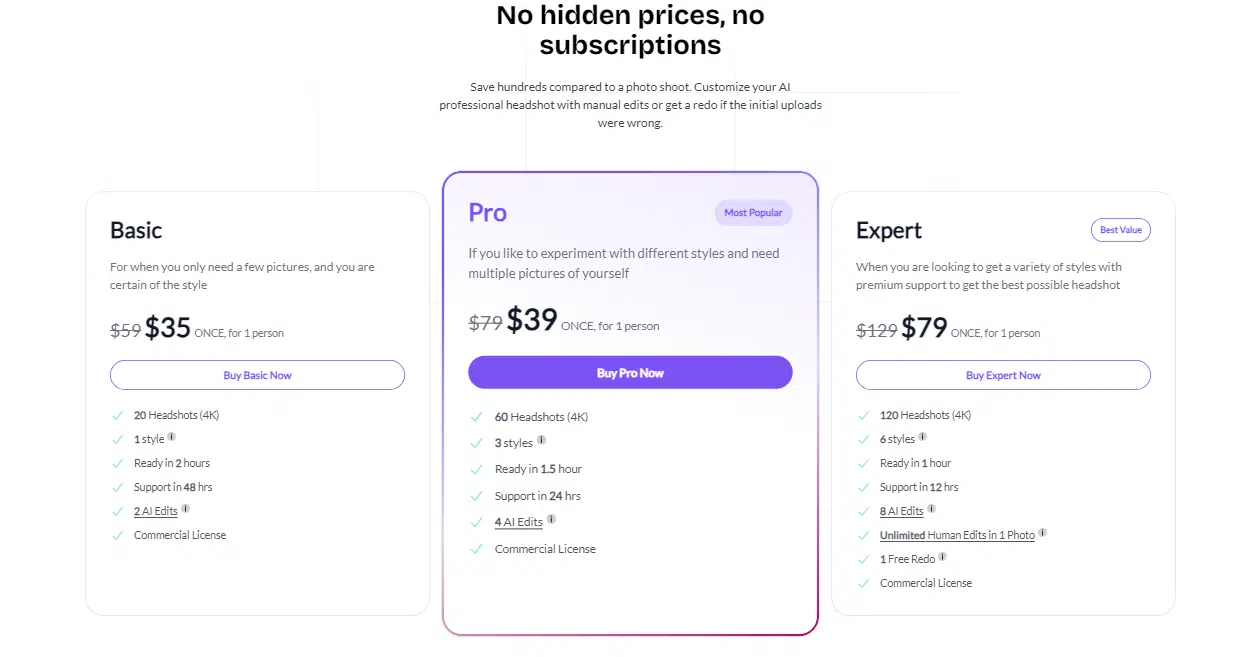
But here’s the catch: all that customization isn’t free. You’ll need credits to make changes, and how many you get depends on which pricing tier you pick (because, of course, we’re back to the trusty three-tiered pricing system).
#8 ProPhotos
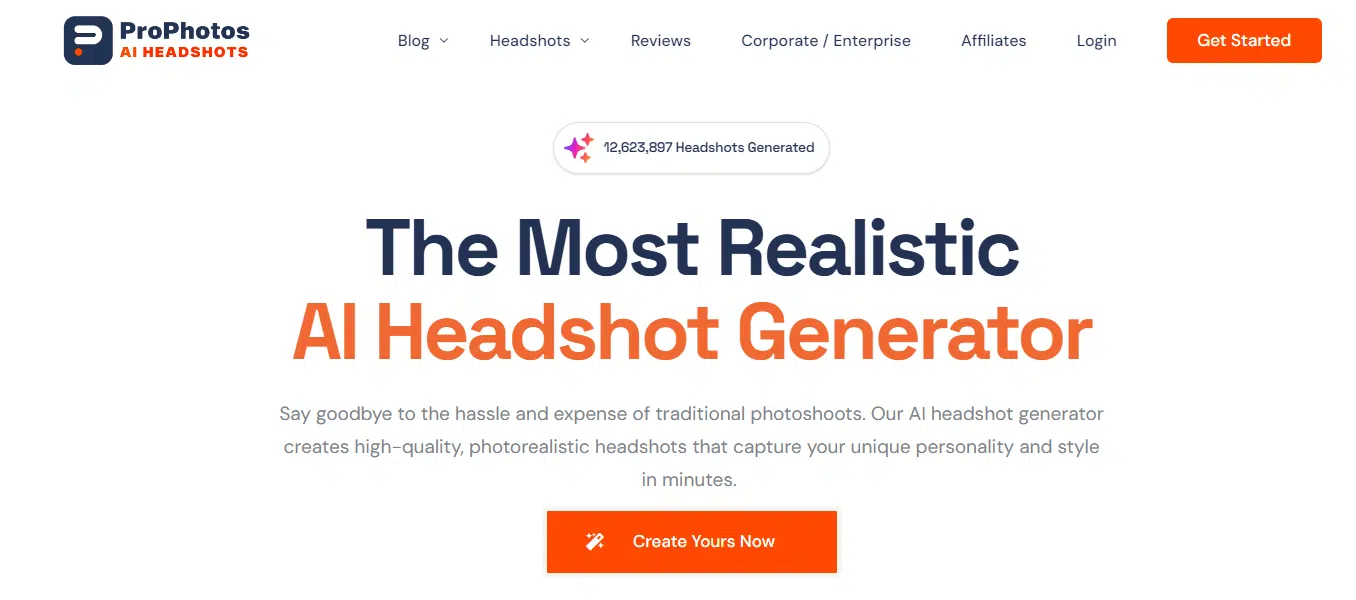
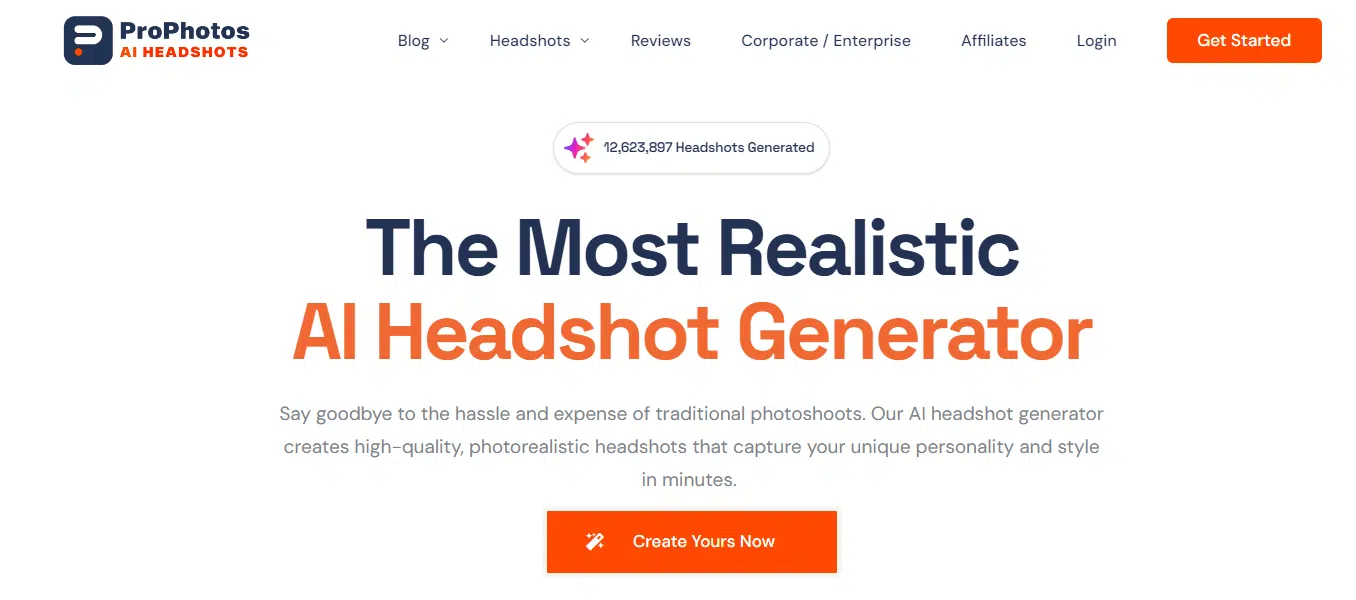
ProPhotos doesn’t quite nail likeness every time, but it still manages to crank out some pretty realistic images. The clothes and faces it generates often look so real you’d have to squint to tell they’re AI-made.
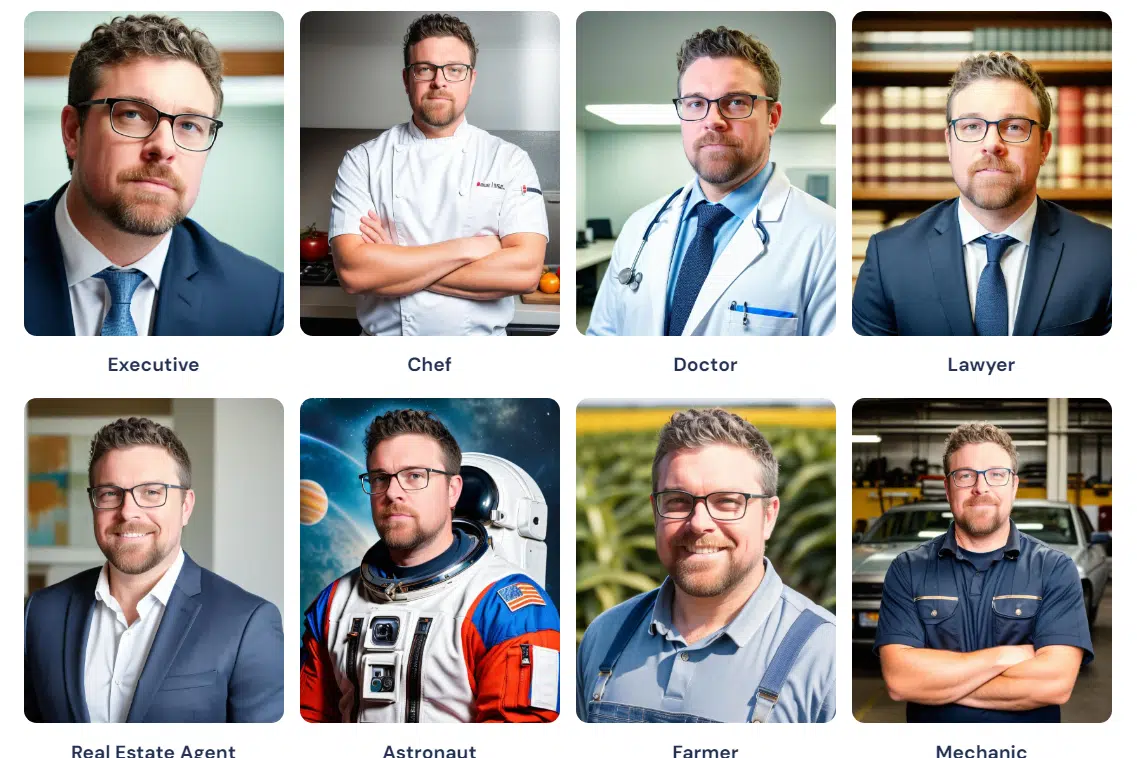
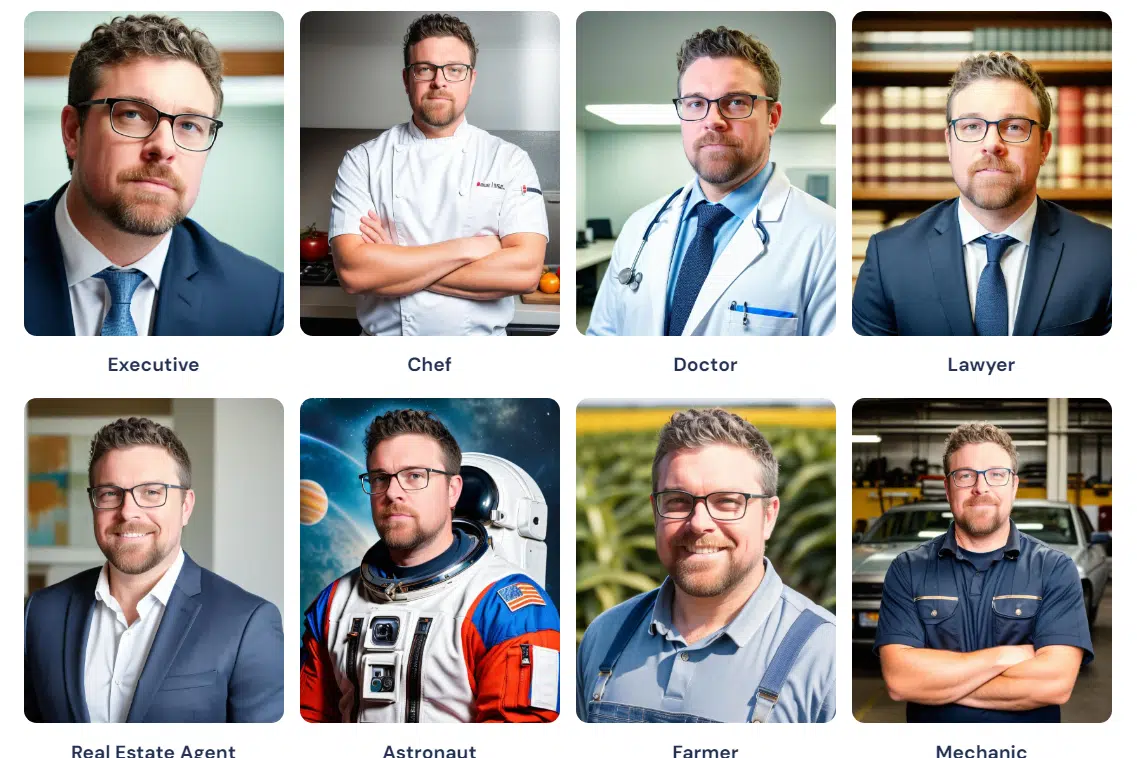
Credits to: ProPhotos
ProPhotos offers a lot of variety. You get different facial expressions, backgrounds, clothing styles, and poses to play with. Out of a typical batch of images, there’s a good chance you’ll find one or two absolute winners.
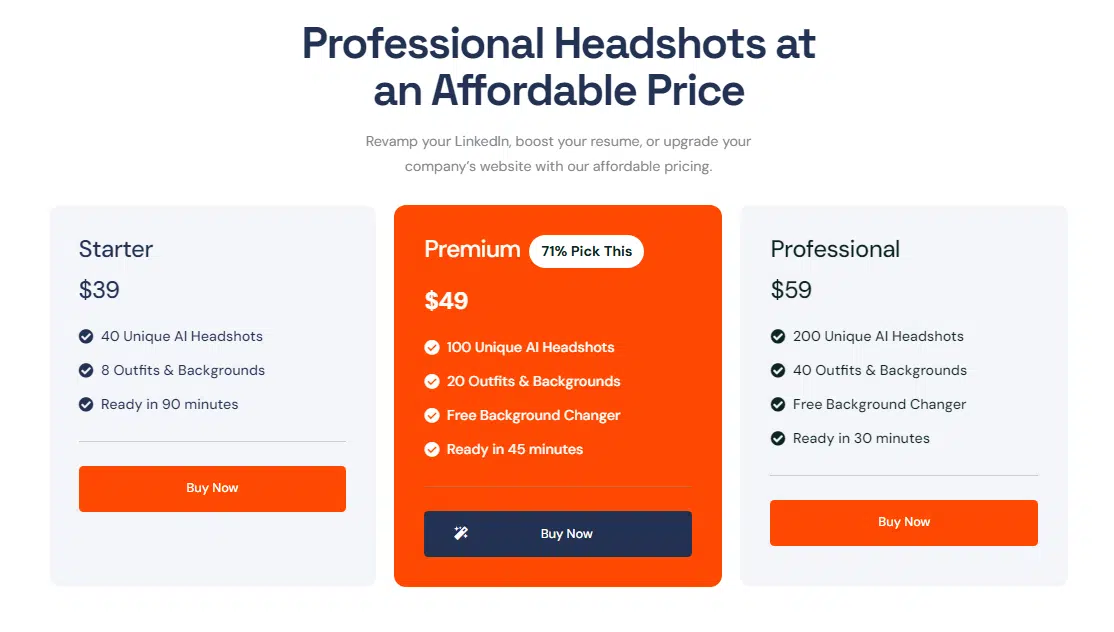
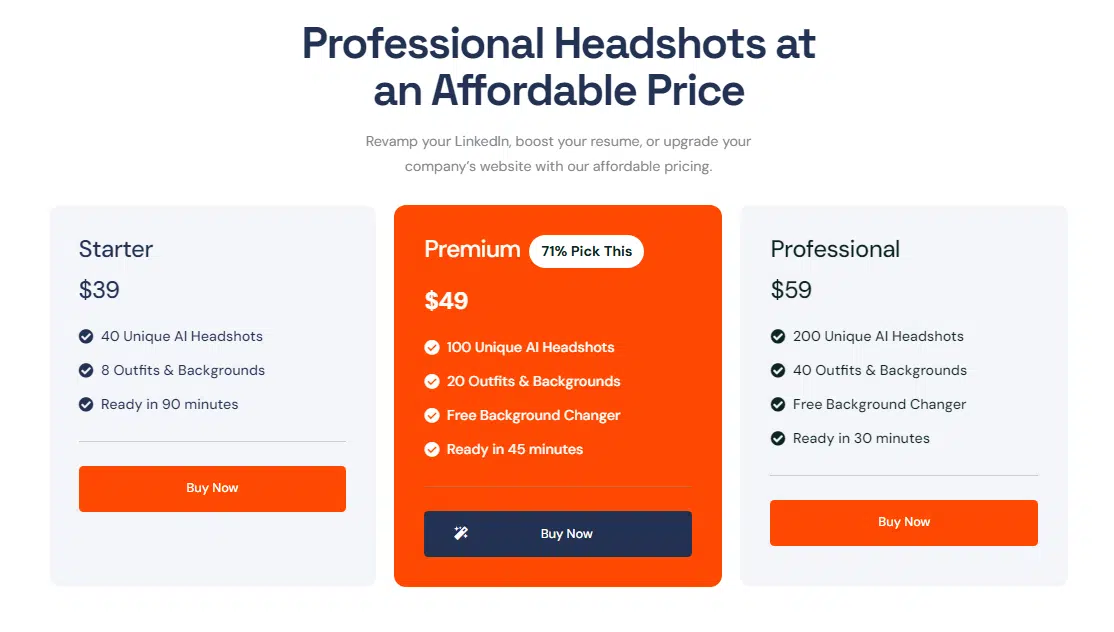
The pricing is what you’d expect—three tiers, like most other generators. Customization isn’t its strong suit, but if you go for the medium or high tiers, you get access to a free background changer, which is a nice touch. At the end of the day, ProPhotos keeps it simple. What you see is what you get—and more often than not, what you get is pretty great.
#9 Dreamwave
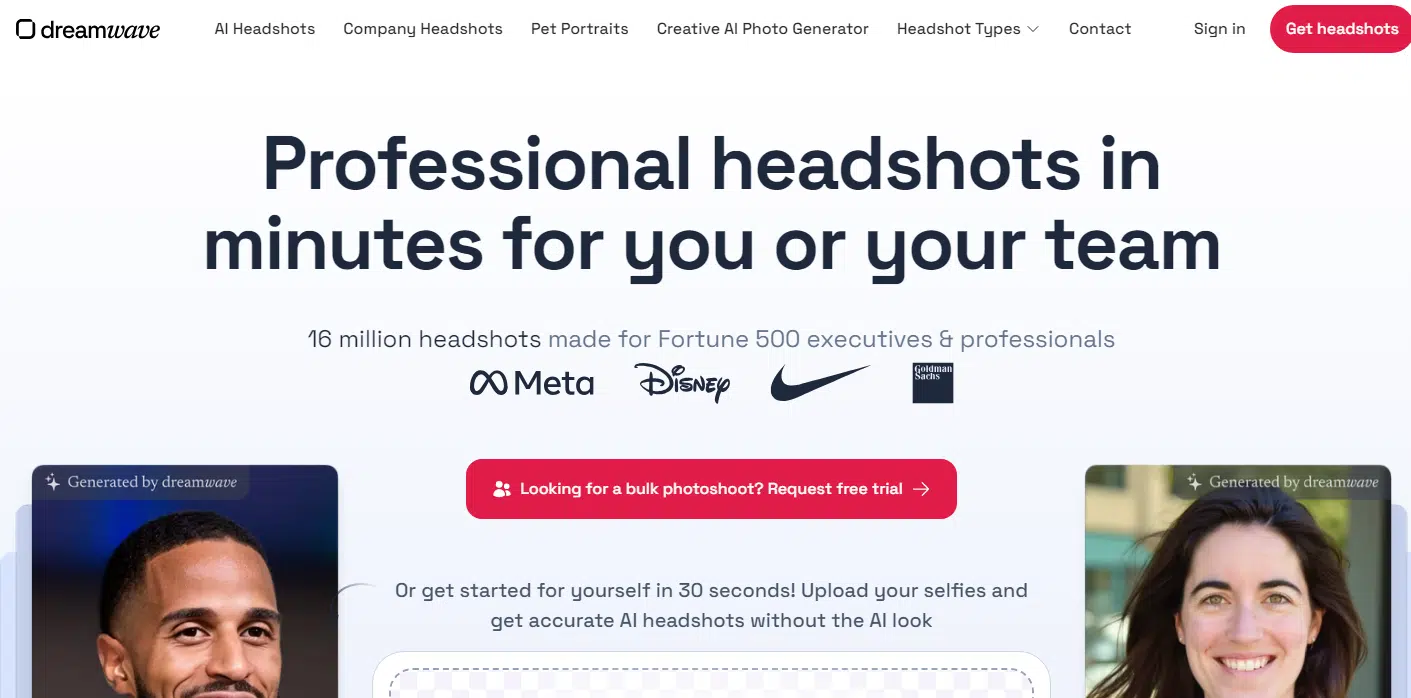
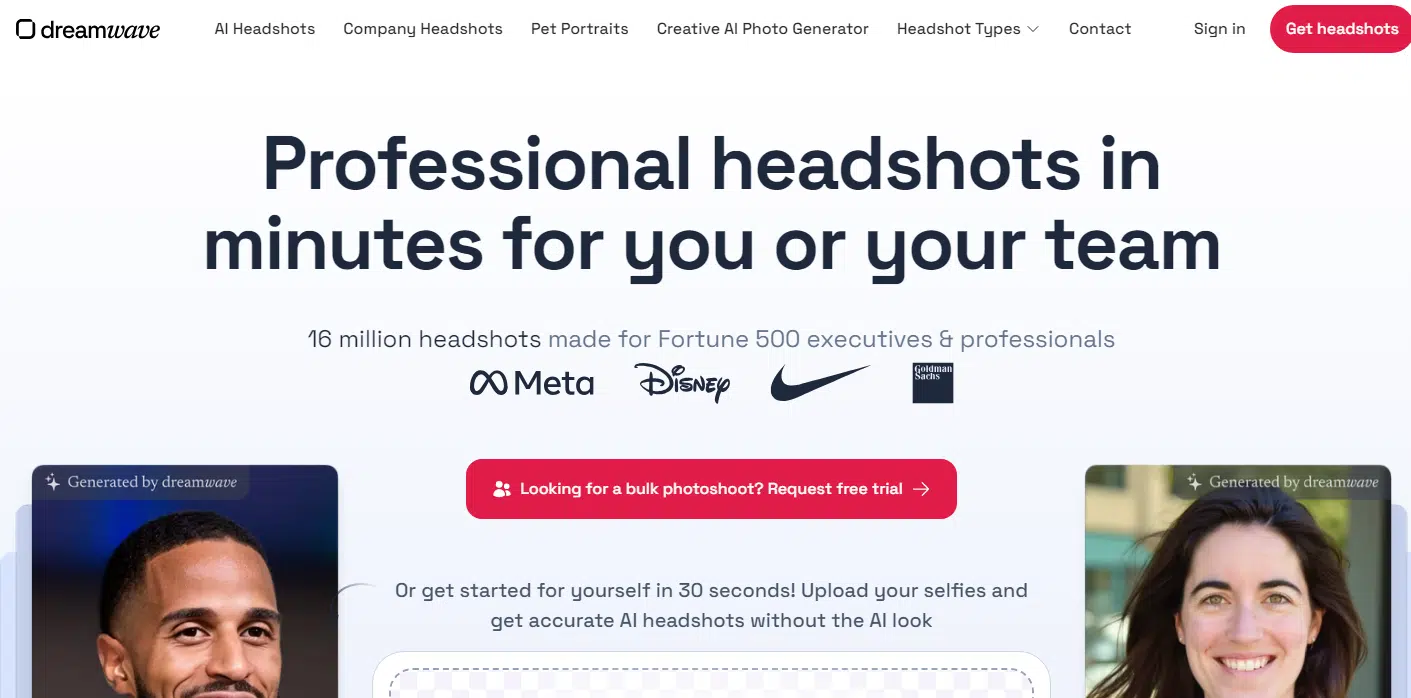
Coming in near the end of this list is Dreamwave, and honestly, it’s a bit of a mixed bag. There were definitely some solid headshots, but overall, my impressions are a little all over the place.
One thing Dreamwave has going for it is its built-in AI image editor. If you’ve got a photo that’s close to perfect but needs some tweaking, you can fix it up and make it work. That’s a nice touch if you’re picky about the final look.
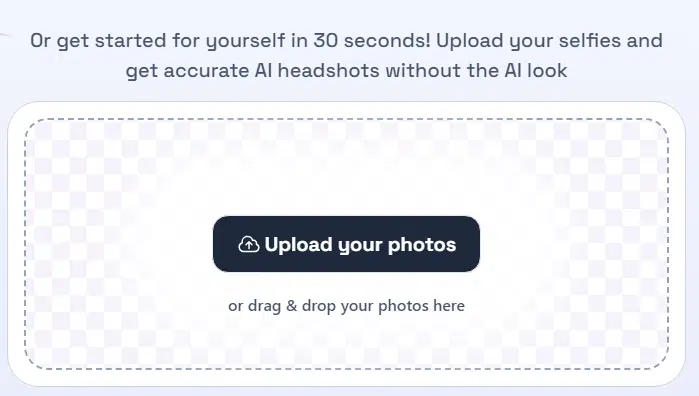
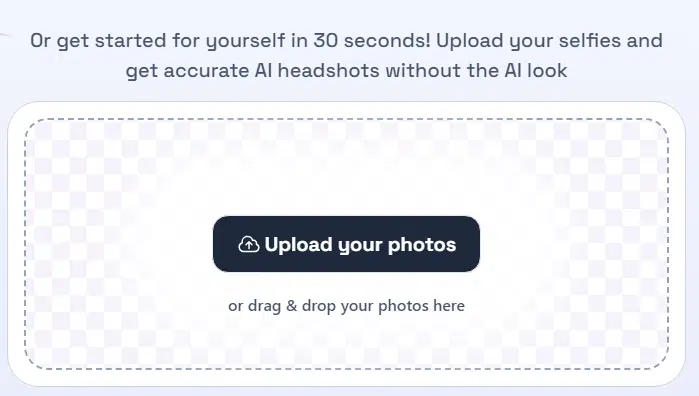
Now, here’s where it gets weird. Dreamwave doesn’t show you pricing upfront—you have to upload your photos first to see the options. Once you do, you’ll find it offers a TON of headshots. The lowest tier gives you 120 images, while the highest throws in 500. Go for the top tier, and you even get unlimited touch-ups by their team of experts. At that point, though, it feels like it’s not really AI-generated anymore.
#10 HeadshotPro
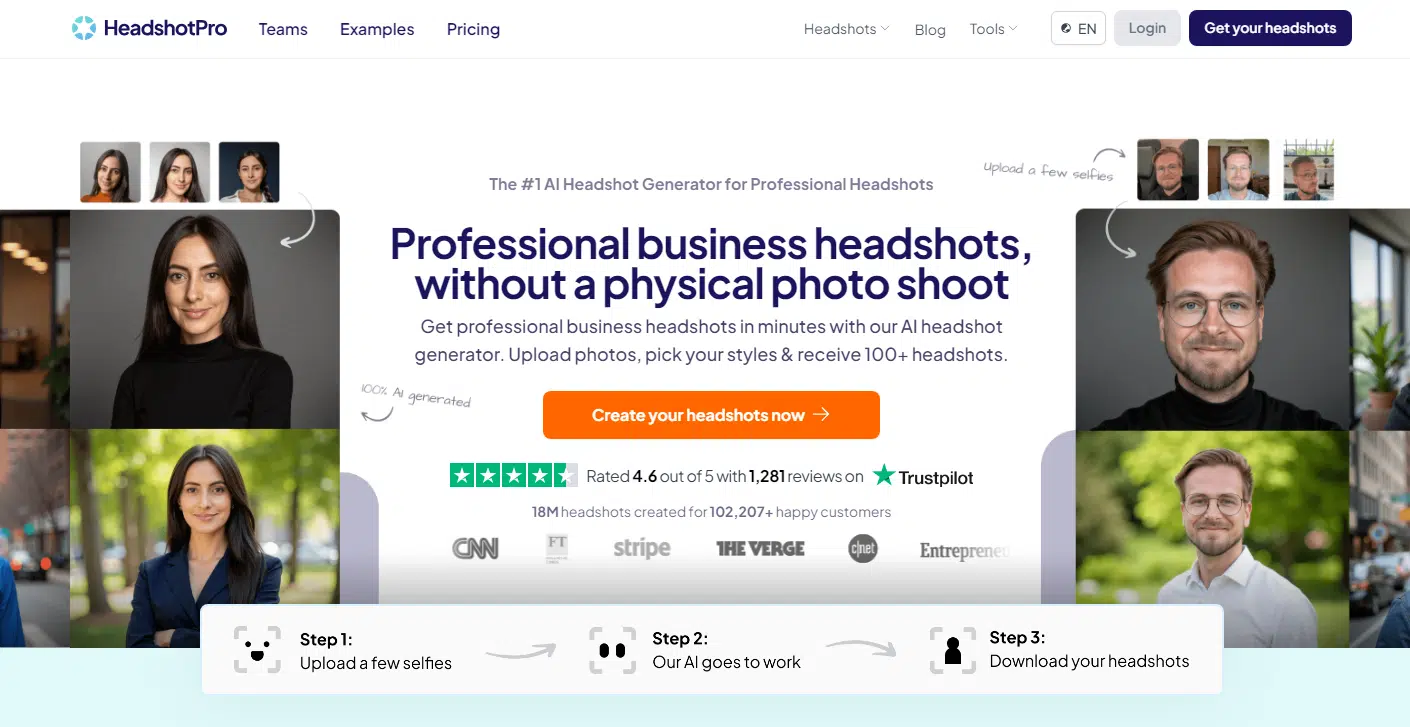
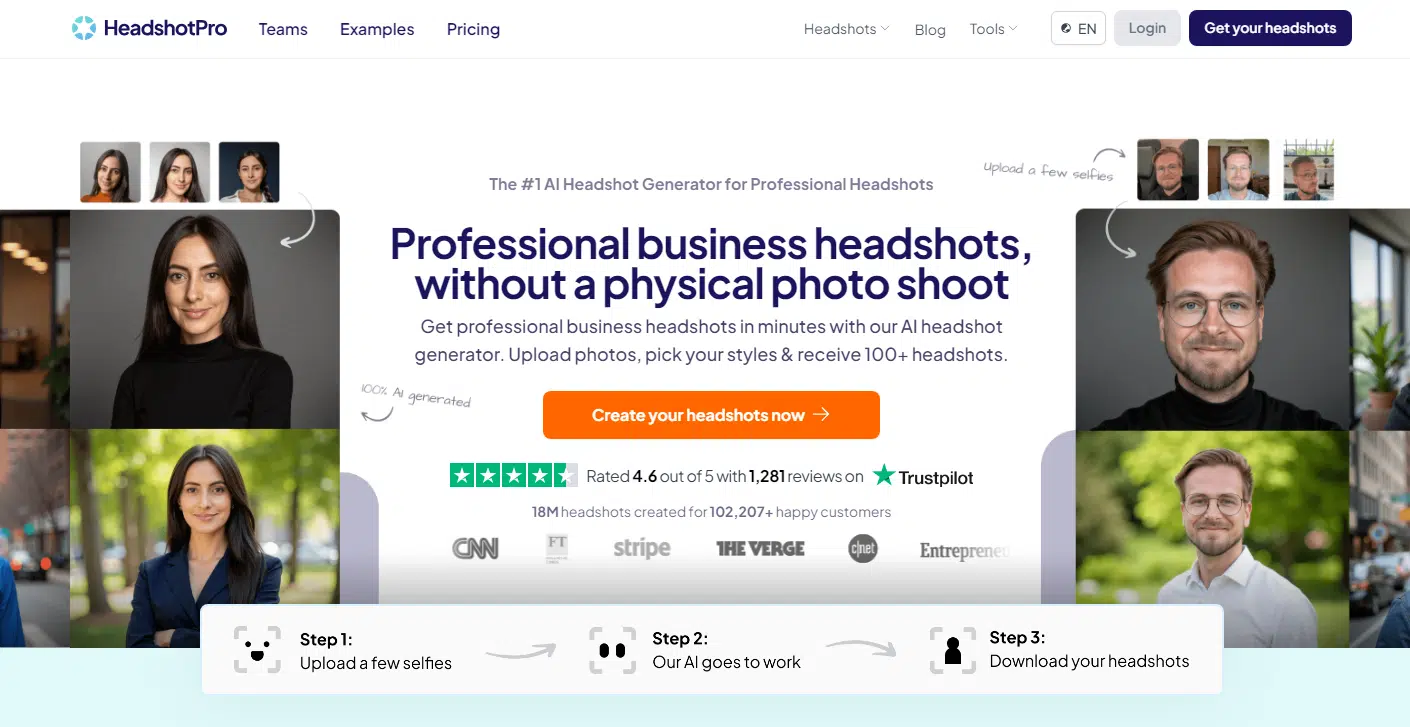
We’ve made it to the final entry on this list, and closing things out is HeadshotPro. For one, it doesn’t quite nail likeness as well as some of the top picks like Portrait Pal. It has a tendency to widen faces, which can look a little off. That said, with a different set of photos—or maybe a bit of AI magic—you might get better results.
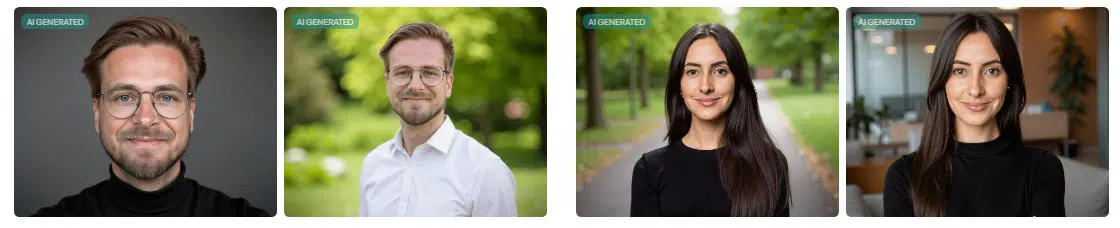
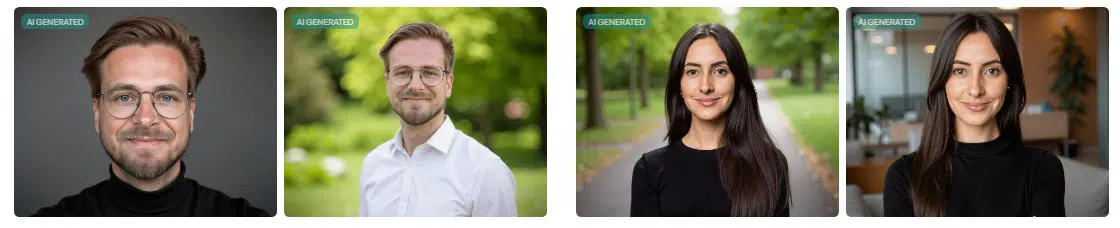
Credits to: HeadshotPro
One of its biggest drawbacks is the “same-face syndrome.” Every headshot comes with the same exact expression, just slightly tweaked in angle or lighting. Sure, there are customization options for poses and backgrounds, but they don’t stray far from the same overall vibe.
On the bright side, the clothing looks pretty spot-on—clean and realistic. The final headshots still come out looking good, but if you’re after variety, this one falls a bit short compared to others on the list.
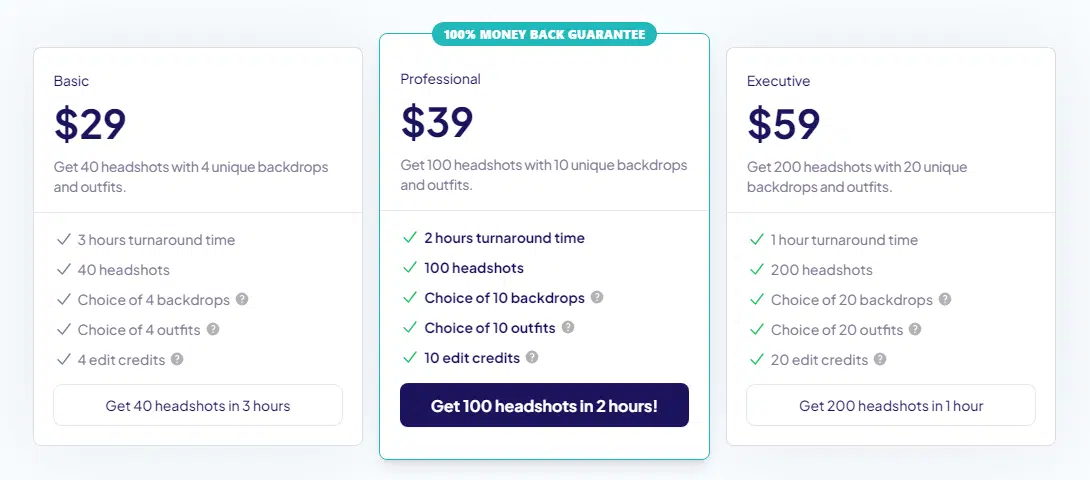
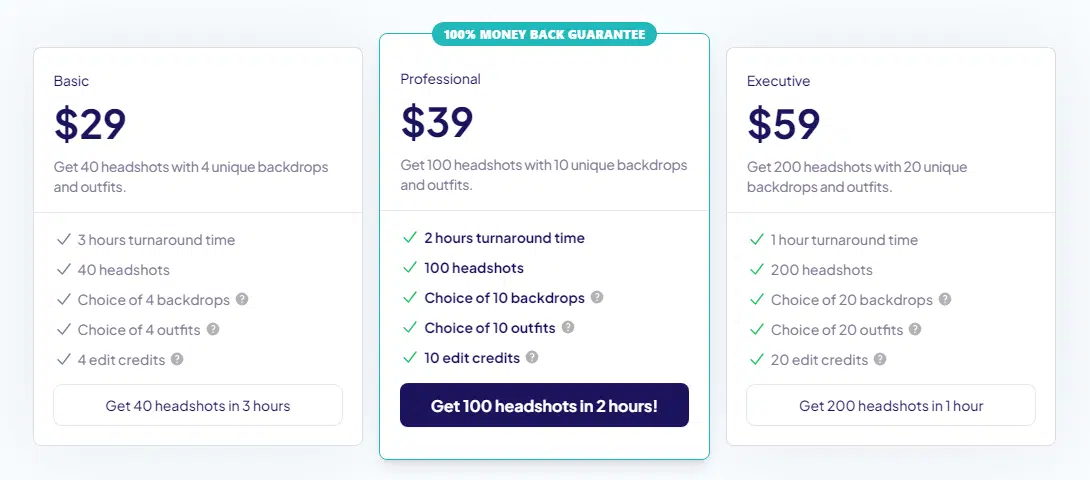
The process itself is straightforward and competitive with other tools. You pick one of three pricing tiers, upload your photos, and get your headshots back within 1 to 3 hours.
My Take on AI Headshot Generators
AI headshot generators have completely changed how we create professional images. They make the whole process faster, more affordable, and way less awkward than booking a photoshoot. If you’re anything like me, the thought of posing in front of a stranger with a camera feels a hassle. These tools take all that stress away—and the results are amazing.
For me, Portrait Pal and PixelPose stood out the most. Both are simple to use, and the results are perfect for anything from LinkedIn profiles to resumes or team pages.
If you haven’t given one of these tools a try yet, now’s the time. All it takes is uploading a few photos, and you’ll have headshots that make you feel more confident about putting yourself out there.
So go ahead—try one out and see what you think. I’m pretty sure you’ll be impressed with the difference it makes!